Anwendung der Nanotechnologie in der Holzwerkstoffindustrie:Ein Rückblick
Zusammenfassung
Die Holzindustrie ist einer der Haupttreiber des Wirtschaftswachstums in Malaysia. Wald als Quelle für verschiedene lignozellulosehaltige Materialien birgt viele ungenutzte Potenziale, die genutzt werden könnten, um nachhaltiges und biologisch abbaubares Nanomaterial herzustellen, das sehr interessante Eigenschaften für den Einsatz in der Holzindustrie selbst oder in vielen verschiedenen Anwendungsbereichen besitzt. Der Sektor Holzprodukte könnte auch verschiedene leicht verfügbare Nanomaterialien verwenden, um die Leistung bestehender Produkte zu verbessern oder neue wertschöpfende Produkte aus dem Wald zu schaffen. Diese Übersicht beleuchtet die jüngsten Entwicklungen bei der Anwendung der Nanotechnologie in der Holzwerkstoffindustrie.
Einführung
Nanotechnologie ist definiert als die Manipulation von Materie zwischen 1 und 100 nm. Die Nanotechnologie ist ein multidisziplinäres Gebiet, das Wissenschaft und Technologie mit dem Ziel verbindet, neue und verbesserte Materialien mit signifikanten Funktionen, physikalischen und chemischen Eigenschaften zu entwickeln [1, 2]. Material dieser Größenordnung hat im Vergleich zu den gleichen Materialien in höheren Dimensionen einzigartige Eigenschaften [3]. Aus diesem Grund können in vielen Disziplinen verschiedene innovative Anwendungen der Nanotechnologie erforscht werden.
Nanotechnologie kann nicht nur als Technologie-Enabler auch ein Treiber für das nationale Wirtschaftswachstum sein. Die Forstindustrie in Malaysia trägt im Jahr 2019 mit einem Wert von rund 22,5 Milliarden RM zum Export bei [4]. Zu den aus Malaysia exportierten Holzprodukten zählen Schnitthölzer, Furniere, Sperrholz und Formteile. Die Holzindustrie in Malaysia hat die Möglichkeit, die Nanotechnologie voll und ganz zu ihren eigenen Vorteilen auszuschöpfen. Es ist unbedingt erforderlich, dass die Bemühungen zur Anwendung der Nanotechnologie in der Holzindustrie, insbesondere in Malaysia, unterstützt und beachtet werden, um die bestehenden Holzprodukte zu diversifizieren und ihren Wert zu steigern, was letztendlich das Wirtschaftswachstum in diesem Bereich ankurbeln wird.
Es ist nicht auszuschließen, dass der Wald seit so vielen Jahren eine wesentliche Rolle für die Zivilisation spielt, da er zur Quelle lignozellulosehaltiger Materialien für verschiedene Produktnutzungen und -entwicklungen geworden ist. Tatsächlich sind Holzprodukte in Form von Hölzern, Möbeln, Papieren und vielen anderen Funktionsmaterialien in der Gesellschaft längst verfügbar [5, 6]. Die Anwendung der Nanotechnologie in der Forstwirtschaft, insbesondere in Forstprodukten oder Holzprodukten, erfordert die Aufmerksamkeit aller beteiligten Akteure und Interessengruppen. Die Nutzung der Nanotechnologie könnte zu stärkeren, multifunktionalen und dennoch leichteren Holzprodukten führen [3]. Traditionelle Forstprodukte wie Zellstoff und Papier, Holzverbundwerkstoffe, Holzbeschichtungen und Holzschutzmittel können erweitert oder in neue oder hochwertige Produkte umgewandelt werden, um breitere und/oder fortschrittlichere Anwendungen zu finden [5].
In diesem Übersichtspapier kann die Anwendung der Nanotechnologie in der Holzwerkstoffindustrie in zwei [2] Pfade unterteilt werden:
- 1
Gewinnung von Nanomaterial(en) aus dem Wald
Zunehmende Bedenken hinsichtlich der Umwelt haben einen immensen Bedarf an der Einführung nachhaltiger und biologisch abbaubarer Materialien in Nanogröße ausgelöst. Dieses neue Material namens Nanocellulose kann auf sichere und nachhaltige Weise aus Waldressourcen hergestellt werden. Der Überfluss an lignozellulosehaltigem Material aus dem Wald hat ein wachsendes Interesse an der Umwandlung von Zellulose in Nanozellulose geschaffen. Nanocellulose ist hauptsächlich Cellulose auf molekularer Ebene, die mehrere Schlüsselmerkmale wie hohe Festigkeit und Steifigkeit, hohes Festigkeits-Gewichts-Verhältnis, elektromagnetische Reaktion und eine große Oberfläche aufweist [5, 7,8,9]. Die herausragenden Eigenschaften dieses Materials bieten enorme Anwendungspotenziale über die Forstproduktindustrie hinaus. Im holzbasierten Produktbereich kann es als Verstärkungsmittel in Zellstoff und Papier und Holzverbundwerkstoffen oder als Beschichtungsmaterial in Holzbeschichtungen verwendet werden [10,11,12]. Aufgrund ihrer Vielseitigkeit könnte Nanocellulose als eine der Komponenten (als Substrat, Stabilisator, Elektrode) für Nicht-Forstprodukte wie Elektronik, Sensoren, Batterien, Lebensmittel, Pharmazie und Kosmetik verwendet werden [5, 13].
- 2
Verwendung von Nanomaterial(en) für holzbasierte Produkte
Nanomaterialien könnten genutzt werden, um bestehende Holzprodukte hinsichtlich ihrer Funktionalität zu verbessern. So kann beispielsweise der Einsatz von Nanomaterialien in der Holzbeschichtung wie Nanozinkoxid oder Nanotitanoxid die Funktionalität des Holzes hinsichtlich Dauerhaftigkeit, Feuerbeständigkeit und UV-Absorption sowie Verringerung der Wasseraufnahme verbessern [14,15,16]. Andererseits könnte die Anwendung der Nanoverkapselung in Holzschutzmitteln [17, 18] die Imprägnierung von Holz mit Pestiziden verbessern, indem dafür gesorgt wird, dass Chemikalien tiefer in das Holz eindringen können und somit das Problem einer übermäßigen Auswaschung reduziert wird [19]. Dies verbessert die Beständigkeit behandelter Hölzer gegenüber biologischen Abbaumitteln.
Es wurden eine Reihe von Übersichtsartikeln zur Anwendung der Nanotechnologie in der Forstwirtschaft und Forstprodukten veröffentlicht [20, 21]. Ein Artikel von McCrank [21] gibt einen Gesamtüberblick über die Anwendung der Nanotechnologie im Forstsektor, während Moon et al. [20] diskutieren mehr über Nanoindentation. Nach bestem Wissen der Autoren gibt es zu diesem Thema nur wenige Veröffentlichungen, die insbesondere ausgewählte Sektoren der Holzindustrie abdecken. Dieses Übersichtspapier wird einen Überblick über die jüngsten Fortschritte bei Nanotechnologieanwendungen in ausgewählten holzbasierten Sektoren geben, nämlich Zellstoff und Papier, Holzverbundwerkstoffe, Holzbeschichtung und Holzbeständigkeit. Darüber hinaus werden einige potenzielle Anwendungen von Nanocellulose als einer neuen Generation von Cellulose in der Holzwerkstoffindustrie in Bereichen wie Energie und Sensorik hervorgehoben.
Anwendungen
Zellstoff und Papier
Die Jahresproduktion von Papier und Pappe beträgt weltweit mehr als 400 Millionen Tonnen [22]. Es zeigt, dass das gegenwärtige digitale Zeitalter die kontinuierliche Verwendung von Papierprodukten in der Gemeinschaft nicht aufgehalten hat. Dennoch besteht die steigende Nachfrage vor allem nach verschiedenen Verpackungsprodukten [23]. Dieser Anstieg könnte durch die Verschiebung der Verbraucherpräferenzen gegenüber dem Online-Shopping und den Aufschwung des E-Commerce begünstigt werden, der es so bequem macht, bequem von zu Hause aus einzukaufen und das Produkt sicher an die vorgesehenen Empfänger geliefert wird.
Papier wird aus lignozellulosehaltigen Materialien hergestellt, die einer Reihe von Primärverarbeitungen, Behandlungen, Papierherstellungen, Trocknungen und Beschichtungen unterzogen wurden. In der Papierherstellungsphase wird das gesamte Mischen von Zellstoff und Zusatzstoffen hinzugefügt und an unterschiedliche Endprodukte angepasst. Nanotechnologie wird bei Zellstoff und Papier in Form von Nanomaterialien oder Nanoadditiven angewendet. Beispielsweise können Waldressourcen oder besser lignozellulosehaltige Materialien genutzt werden, um den nanoskaligen Baustein oder die sogenannte Nanozellulose zu gewinnen, die als Verstärkungseinheit dient, um eine einzigartige mechanische Festigkeit, Funktionalität und Flexibilität bereitzustellen [5]. Diese neue Generation von Zellulose wird durch Zellwanddelamination zur Gewinnung von Nanofibrillen oder durch Extraktion von kristalliner Zellulose im Nanomaßstab hergestellt. Nanocellulose wird der Papierherstellung hauptsächlich zugesetzt, um die Papierfestigkeit zu erhöhen. Andere Nanomaterialien, die während des Papierherstellungsprozesses verwendet werden, umfassen Nanosilica, Nanozeolith usw. [22]. Die Einarbeitung von Nanomaterialien oder Nanoadditiven in die Papierindustrie kann dazu beitragen, die Leistung der Papierprodukte zu verbessern.
Die wichtigsten Additive, die in der Zellstoff- und Papierindustrie verwendet werden oder möglicherweise verwendet werden können, werden in den folgenden Abschnitten erörtert.
Nanocellulose als Nass- oder Trockenfestigkeitsmittel
Nanocellulose wurde als Festigkeitsadditiv im Zellstoff- und Papierbereich umfassend untersucht [24, 25]. Nanocellulose stößt aufgrund ihrer Häufigkeit, Verfügbarkeit und auch wegen ihrer interessanten Eigenschaften wie biologisch abbaubar, geringe Toxizität, hervorragende mechanische und optische Eigenschaften, hohe Oberfläche und Erneuerbarkeit auf enormes Interesse [5, 7]. Nanozellulose ist eigentlich Zellulose im Nanomaßstab, die aus allen lignozellulosehaltigen Materialien isoliert und hergestellt werden kann, einschließlich Zellstoff, Nichtholzpflanzen, Holz und landwirtschaftlichen Rückständen, Bakterienzellulose und Manteltieren. Cellulose ist eine lineare Verbindung mit β-1,4-verknüpften Glucoseeinheiten, die kristalline und amorphe Bereiche umfasst. Die Entfernung amorpher Domänen aus der Zellulosekette mit Mineralsäure führt zur Isolierung nanokristalliner Zellulose, während die Zellwanddelamination durch mechanische Einwirkung hoher Scherkräfte zu einer Verringerung der Zellulosebreite führt, wodurch nanofibrillierte Zellulose entsteht. Diese beiden Kategorien von Nanocellulose sind die am besten untersuchten Nanomaterialien im Zellstoff- und Papierbereich zur Produktverbesserung. In den letzten zehn Jahren konzentrierten sich zahlreiche Arbeiten auf die Herstellung von nanofibrillierter Cellulose (NFC) und nanokristalliner Cellulose (NCC) aus verschiedenen Pflanzenressourcen. Abbildung 1 zeigt Bilder von nanokristalliner Cellulose und nanofibrillierter Cellulose mit Transmissionselektronenmikroskopie (TEM).
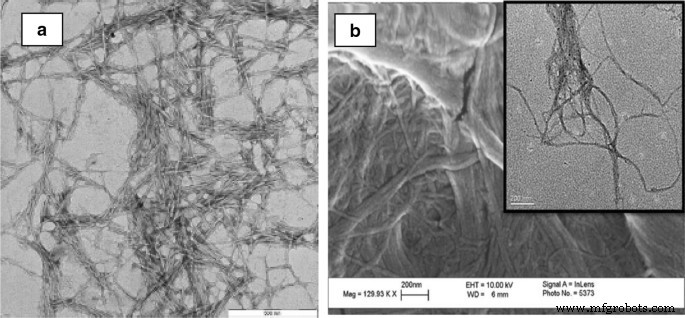
TEM-Aufnahme von NCC von Acacia Magium a Quelle :Jasmani und Adnan [26]. Reproduziert mit Genehmigung von Elsevier und NFC aus Nadelholzzellstoff, b Quelle :Zhaoet al. [27]. Reproduziert mit Genehmigung von Elsevier
Die Eigenschaften von Nanocellulose variieren je nach Herstellungsverfahren und Cellulosequelle. Tabelle 1 zeigt die Morphologie und Kristallinität von nanofibrillierter Cellulose und nanokristalliner Cellulose.
Von Zellulose zu Nanozellulose
Nanofibrillierte Cellulose ist das am besten untersuchte Nanoadditiv in der Papierherstellung, da über viele Arbeiten berichtet wurde [3, 25]. Nanofibrillierte Cellulose wird hergestellt, indem Fasern selektiven mechanischen Behandlungen mit hoher Scherung unterzogen werden. Es gibt viele Verfahren und Kombinationen von Verfahren, um nanofibrillierte Cellulose herzustellen. Homogenisierung und Mikrofluidisierung sind die üblichen Techniken, die für die Herstellung von nanofibrillierter Cellulose verwendet werden, bei der die Fasern in einer kleinen Düse unter hohem Druck für wiederholte Durchgänge in der Regel zwischen 10 und 15 Durchgängen ausgesetzt werden [32, 33]. Diese Art der Behandlung erfordert allein einen hohen Energieverbrauch, daher eine Vorbehandlung mit Chemikalien wie TEMPO (2,2,6,6-Tetramethylpiperidin-1-oxyl)-vermittelte Oxidation [34], Carboxymethylierung oder Enzym [35, 36 ] wird normalerweise benötigt, um Energie zu sparen. Bei diesem Verfahren wird die nanofibrillierte Cellulose als Ergebnis hoher Scherraten erzeugt, die durch die hohe Geschwindigkeit und Kraft des Fluidstroms induziert werden. Ein weiteres beliebtes Verfahren zur Herstellung von nanofibrillierter Cellulose ist das Mahlverfahren. Die Schleifmaschine besteht aus statischen und rotierenden Steinscheiben. Die von den Steinen erzeugte Scherkraft zerlegt die Zellwandfasern zu vereinzelten Nanofibrillen. Um bei dieser Methode eine breite Breitenverteilung aufgrund von Aggregation zu vermeiden [37], empfahlen die Autoren, die Einsatzstoffe nach Aufschluss- und Bleichprozessen in Wasser zu halten. Dies soll die Bildung von Wasserstoffbrückennetzwerken im trockenen Zustand verhindern [37, 38]. Das Ultraschallverfahren wurde auch untersucht, um Nanofasern herzustellen. Bei der Beschallung wird eine hohe Kavitationsscherkraft durch Ultraschallwellen erzeugt, die die Faserzellstruktur aufbrechen kann [39]. Die Beschallungstechnik soll gut dispergierte und stabile Nanofasern erzeugen [3]. Darüber hinaus kann Kryo-Crushing auch angewendet werden, um native Fasern in Nanofasern umzuwandeln. Die Fasern müssen mit flüssigem Stickstoff eingefroren werden, gefolgt von hohen Scherkräften. Die hohe Scherkraft induzierte einen Druck auf die Eiskristalle, der die Zellwände dazu zwingt, aufzubrechen und die Mikrofibrillen freizusetzen [40,41,42,43].
Aus nativer Cellulose extrahierte nanokristalline Cellulose wird üblicherweise durch Säurehydrolyse hergestellt. Andere Methoden, die den Einsatz spezifischer Chemikalien beinhalten, wie TEMPO-vermittelte Oxidation [44] und ionische Flüssigkeiten [45] wurden ebenfalls für die Herstellung von Nanocellulose untersucht. Auch über eine Anwendung von Cellulase-Enzym wurde berichtet [46, 47].
Während der sauren Hydrolyse nimmt der Polymerisationsgrad von Cellulose schnell ab, stabilisiert sich jedoch irgendwann, dem sogenannten Level-Off-Polymerisationsgrad [48]. Der Grund für dieses Verhalten könnte darin liegen, dass amorphe Bereiche durch die Säure schnell hydrolysiert werden. Zu Beginn der Hydrolyse greift Säure aufgrund ihres hohen Volumens bevorzugt den amorphen Bereich an und hydrolysiert die leicht zugängliche glykosidische Bindung [49]. Sobald die leicht zugängliche Bindung hydrolysiert ist, erfolgt die weitere Hydrolyse am reduzierenden Ende der Glucosekette und auf der Oberfläche des kristallinen Bereichs viel langsamer [50]. Die Säurehydrolyse wird durch Faktoren wie Säuretyp, Säurekonzentration, Temperatur und Zeit beeinflusst [49, 51, 52]. Jede Änderung der Säurekonzentration, Hydrolysezeit und Temperaturbedingung hat einen Einfluss auf die Morphologie der nanokristallinen Cellulose. Beispielsweise führt eine Erhöhung sowohl der Hydrolysezeit [53] als auch der Säurekonzentration [29] zu einer kürzeren nanokristallinen Cellulose, während eine hohe Temperatur zu einer vollständigen Umwandlung von Cellulose in Glucose führt [50, 54, 55].
Die Zugabe von Nanocellulose bei der Papierherstellung führt zu einem Papier mit einer besseren Leistung, indem es die Papierfestigkeit [56, 57] und die Dichte [58, 59, 60] erhöht und auch die Porosität verringert [61]. Die Zugabe von nanostrukturierter Cellulose führt zu ähnlichen Papiereigenschaften mit gemahlenen Fasern [3, 25]. Theoretisch kann die Festigkeit von Papier durch Zugabe von Nass- und Trockenfestmitteln [24, 62], Zugabe von funktionalisierten Fasern [63] und Mahlen [64] erhöht werden. Die Verbesserung führt zu einer Faserbindungsfähigkeit [25]. Boufiet al. [25] schlugen vor, dass der Mechanismus auf die Zunahme der gebundenen Fläche zurückzuführen ist, die darauf zurückzuführen ist, dass Nanozellulose als ein Verbindungselement zwischen den Fasern fungiert, das zu einer Faser-zu-Faser-Bindung führt. Darüber hinaus kann es auch an unterschiedlichen Netzwerken liegen, die in der Faser erzeugt werden, die zu einer Erhöhung der Bindungsfähigkeit führen. Aufgrund der Mikrometerlänge von Nanocellulose können sie als Brücke fungieren, um die benachbarten Fasern zu verbinden, was zu einem stärkeren Netzwerk führt [25]. Das wechselseitige Bindungsnetzwerk von Fasern und Nanozellulose erhöht die Festigkeit des Papiers.
Nanocellulose als Nanoadditiv verbessert die interne Bindung, was zu einer Erhöhung der Trockenzugfestigkeit, einer Verringerung der Luftdurchlässigkeit und Opazität und einer höheren Dichte führt [3]. Als nanoskaliges Material hat Nanocellulose eine große Oberfläche und somit können Wasserstoffbrückenbindungen effektiver gebildet werden. Es wird als Wet-End-Additiv zur Verbesserung der Trockenfestigkeit und Retention hinzugefügt. Nanocellulose, die üblicherweise als Trockenfestigkeitsadditiv verwendet wird, liegt in Form von nanofibrillierter Cellulose vor.
Es gibt verschiedene Strategien, NFC in den Faserstoffeintrag zu geben, da es direkt mit [24, 62, 65] oder ohne Retentionshilfe [58] oder gemischt mit anderen Füllstoffen oder Langfasern [66, 67] gefolgt von einer Retentionshilfe zugegeben werden kann . Eine Erhöhung der Zugfestigkeit nach NFC-Zugabe bezieht sich auf die Zugabemenge. Es wurde festgestellt, dass eine Zunahme der Zugfestigkeit von 5 % beobachtet wurde, wenn 3 % NFC in den Faserstoffeintrag aus gemahlenem Faserstoff zusammen mit kationischer Stärke zugegeben wurden [24]. Es ist interessant festzustellen, dass der Effekt bemerkenswerter war, wenn das Fruchtfleisch weniger geschlagen wurde. Beispielsweise erhöhte sich die Zugfestigkeit um mehr als 100 %, wenn dem thermomechanischen Zellstoff 6 % NFC zugesetzt wurden. Auf der anderen Seite hatte NFC eine geringere Wirkung, wenn es gut geschlagenem Zellstoff zugesetzt wurde [65]. Daher wird erwartet, dass eine Erhöhung der Zugfestigkeit auftritt, wenn NFC zu gefülltem Papier, mechanischem Zellstoff und recyceltem Zellstoff hinzugefügt wird. Hi et al. [65] berichteten über die Beobachtung, dass NFC auf dem Füllstoff und den Fasern adsorbiert wird und den Füllstoff mit dem Fasernetzwerk überbrückt. Der einzige große Nachteil bei der Verwendung von NFC bei der Papierherstellung besteht darin, dass es die Entwässerung verlangsamt. Die Entwässerung spielt bei der Papierherstellung eine sehr wichtige Rolle, da sie direkt mit der Effizienz der Papierherstellung zusammenhängt. Je langsamer die Entwässerungszeit, desto langsamer wird das Papier produziert. Daher ist es sehr wichtig, Retentionsmittel in einer bestimmten Dosierung zu verwenden, um die Adsorption der Nanofasern an der Faseroberfläche zu verbessern, was zu einer verbesserten Entwässerung führt [24, 68].
NFC hat Eigenschaften zwischen Trockenfestigkeitsadditiv wie Stärke und Feinkorn, das durch Schlagen entsteht, da beide zu einer Erhöhung der gebundenen Fläche führen [3]. Dies wird erreicht, indem eine weiche und dünne Schicht auf der Faseroberfläche erzeugt wird, die dazu beiträgt, die Faserbindung während des Trocknens zu erhöhen, und auch durch das Auffüllen von Hohlräumen und Poren zwischen den Fasern, die auch die gebundene Fläche erhöhen [3].
Nanocellulose als Beschichtungsmaterial für Barriereeigenschaften
Nanocellulose kann auch als Beschichtungsmaterial in Verpackungspapier verwendet werden, da es gute Barriereeigenschaften besitzt. Der Vorteil der Verwendung von Nanocellulose als Beschichtungselement besteht darin, dass das Entwässerungsproblem kein Thema mehr ist, da es nach der Papierherstellung zugegeben wird. Es gibt verschiedene Ansätze, die zum Auftragen von Nanocellulose verwendet werden können, darunter Sprühen, Stabbeschichtung, Leimpressen und Walzenbeschichtung. Es wurde berichtet, dass die Anwendung von Nanocellulose, insbesondere nanofibrillierter Cellulose, die Sauerstoffbarriere und die Ölbeständigkeit erhöht [69]. In einem Beispiel wurde eine Verringerung der Luftdurchlässigkeit von 69.000 auf 4,8 und 660 auf 0,2 nm Pa −1 . beobachtet für ungebleichtes Papier bzw. fettdichtes Papier [70] unter Verwendung des Stabbeschichters. Syverud und Stenius [71] verwendeten unterschiedliche NFC-Mengen von 0 bis 8 % auf Weichholzzellstoff und stellten fest, dass sich die Barriereeigenschaften von 6,5 auf 360 nm Pa −1 . deutlich verbesserten s −1 . Dies liegt an der verringerten Porosität, die durch die Zunahme der Nanofibrillen verursacht wird. Die Anwendung von NFC und Schellack wurde auch von Hult et al. [72] auf Papier und Pappe, was zu einer Verringerung der Luftdurchlässigkeit, der Sauerstoffdurchlässigkeit und der Wasserdampfdurchlässigkeit führt, was es zu einem Potenzial für Barriereverpackungen macht.
Darüber hinaus kann Nanocellulose, insbesondere nanofibrillierte Cellulose, in freistehende dünne Filme oder Nanopapiere umgewandelt werden. Dieses Nanopapier kann als Substrat für elektronische Anwendungen verwendet werden, da es transparent und flexibel ist [73].
Nanomaterial als Retentionsmittel zur Immobilienverbesserung
Retentionsmittel werden der Papierherstellung zugesetzt, um die Retention funktioneller Chemikalien auf Papieren zu verbessern. Einige Nanomaterialien wurden in Papierprodukten getestet, wie die Verwendung von Nanozeolith [74] und Nanotitandioxid. Nanozeolith wird als Trockenmittel in der Papierindustrie verwendet, um Feuchtigkeit zu absorbieren und dient auch zur Entfernung von Gasemissionen, wenn es für Spezialpapier verwendet wird. Die große Oberfläche des Nanozeoliths, bestehend aus Hohlräumen und Poren, hilft während eines solchen Prozesses. Zu Papier hinzugefügtes Nanotitanoxid kann ein Papier mit einem besseren dynamischen Elastizitätsmodul im Vergleich zu einer kontrollierten Probe bilden [75].
Nanofiller-Effekt zur Verbesserung der Eigenschaften
Die Verwendung von Füllstoffen in der Papierindustrie ist hauptsächlich auf Kostensenkungen zurückzuführen, da Füllstoffe in der Regel billiger sind als Zellstoff selbst. Neben der Untersuchung als Festigkeitsadditiv kann Nanocellulose auch als Füllstoff verwendet werden. Die Zugabe von nanofibrillierter Cellulose kann die Holzstoffmenge reduzieren und die Füllstoffmenge erhöhen, wodurch die Produktionskosten gesenkt werden können [76]. Darüber hinaus weist das hergestellte Papier verbesserte Eigenschaften wie geringe Porosität und hohe Opazität auf. Es wurde auch berichtet, dass die Zugabe von 2–10% nanofibrillierter Cellulose als Füllstoff zu einer Festigkeitssteigerung von 50–90% führt (Future Markets Inc. 2012). Nanoclay kann als Additiv bei der Papierherstellung verwendet werden, um die Gasdurchlässigkeit zu verringern, die zu einer längeren Haltbarkeit des Papiers führen kann. Dies ist in der Verpackungsindustrie unerlässlich, in der Gas- und Wasserbarrieren eine wichtige Rolle bei der Verhinderung des Verderbens von Lebensmitteln und Getränken spielen.
Nanocalciumcarbonat wird als Füllstoff zur Verbesserung der Lichtstreuung verwendet. Modifiziertes gefälltes Calciumcarbonat mit nanostrukturierten Partikeln hatte einen positiven Einfluss auf die Lichtstreuung [77]. Ausgefälltes Calciumcarbonat wurde mit Silikat- und Zinksulfid-Nanopartikeln beschichtet. Wildet al. [78] berichteten über eine ähnliche Studie, in der Nanopartikelbeschichtungen in Labor-, Pilot- und Mühlenversuchen verwendet wurden. Die Studie ergab, dass die Beschichtung mit Nanopartikeln eine gute Druckqualität, Wasserbeständigkeit und Dimensionsstabilität bietet. Dem Papierrohstoff zugesetztes Nanozinkoxid verleiht dem Papier antibakterielle Eigenschaften. Gleichzeitig verbesserten sich durch Zugabe von Nanozinkoxid auch die optischen Eigenschaften wie Helligkeit und Weißgrad sowie die Bedruckbarkeit des Papiers. Nanotitanoxid wurde auch in Kombination mit Beta-Cyclodextrin in beschichtetem Papier untersucht [79]. Es wurde festgestellt, dass die Nanomaterialmischung eine bessere Abbauwirkung auf Xylol hatte als nur mit Nanotitanoxid beschichtetes Papier.
Nanomaterial als Schlichtemittel zur Eigenschaftsverbesserung
Der Papierherstellung wird ein Leimungsmittel zugesetzt, um die Beständigkeit gegen das Eindringen von Wasser/Flüssigkeit zu verbessern, so dass das Papier für Druck- und Schreibzwecke geeignet ist. Die Verwendung von Nanosilica kann die optischen Eigenschaften verbessern und den Durchdruck um bis zu 30% reduzieren. Es wurde festgestellt, dass mit Nanosilica beschichtetes Papier eine bessere optische Dichte, Dimensionsstabilität und Druckqualität als unbeschichtetes Papier liefert [80,81,82].
Holzverbundwerkstoffe
Holz ist ein Geschenk der Natur an die Menschheit, da es ein biologisch abbaubarer und nachwachsender Werkstoff ist, der in vielen Anwendungen eingesetzt werden kann. Das Holz selbst weist jedoch mehrere Schwächen auf, wie z. B. zart, nicht flexibel und nicht haltbar aufgrund von Termitenangriffen und anderen. Die Verwendung von Holzfasern zur Herstellung von Holzverbundwerkstoffen hat seine Nachteile, da sie eine geringe Schüttdichte, eine geringe thermische Stabilität, eine hohe Neigung zur Feuchtigkeitsaufnahme und eine Anfälligkeit für biologischen Abbau aufweisen. Nanotechnologie wird in vielen Wissenschaften eingesetzt und kann verwendet werden, um die Qualität vieler Materialien zu verbessern, einschließlich Holz und Holzverbundwerkstoffen.
Nanocellulose als Verstärkungsmaterial
Das Prinzip der Verwendung von Nanocellulose als Verstärkungsmaterial im Matrixmaterial hat zu vielen Forschungsarbeiten geführt. Durch die Zugabe von nanoskaliger Cellulose besitzen die Nanokomposite in vielerlei Hinsicht hervorragende Eigenschaften, die durch Mikrokomposite nicht erreicht werden könnten [83]. Diese nanozelluloseverstärkten Verbundwerkstoffe sind in der Lage, die herkömmlichen Verbundwerkstoffe zu ersetzen. Durch entsprechende Modifikation von NCC können verschiedene funktionelle Nanomaterialien mit herausragenden Eigenschaften oder deutlich verbesserten physikalischen, chemischen, biologischen sowie elektronischen Eigenschaften entwickelt werden. Die Eigenschaften von Nanokompositen hängen von wenigen Faktoren ab, wie den Eigenschaften des Matrixmaterials, den Eigenschaften von Nanocellulose, der Dispersion von Nanocellulose im Matrixmaterial und den Grenzflächenwechselwirkungen zwischen Füllstoff und Matrixmaterial [84].
Nanozellulose als Verstärkung in verschiedenen Typen von Matrixmaterialien wurde umfassend untersucht. Als vielversprechende Materialklasse haben sich Polymer-Nanokomposite aus natürlichen oder synthetischen Polymeren, die mit Nanocellulose verstärkt sind, etabliert. Die Verbesserung der mechanischen Eigenschaften ist das häufigste Ziel bei der Herstellung dieser mit Nanocellulose verstärkten Polymere [85,86,87]. Polymere auf Erdölbasis werden im Allgemeinen in thermoplastische und duroplastische Typen unterteilt. Der Unterschied zwischen thermoplastischen und duroplastischen Polymeren besteht in der Bindung, die ihre langkettigen Moleküle hält, erstere wird durch schwache Van-der-Waals-Bindungen gehalten, während letztere durch starke kovalente Bindungen gehalten wird [88]. Eine Vielzahl von Duroplasten wurde für die Verwendung in Nanocellulose-Kompositen untersucht. Epoxidharz wurde zum Beispiel aufgrund seiner ausgezeichneten Bindungseigenschaften und guten mechanischen Eigenschaften nach dem Aushärten (hoher Modul, geringes Kriechen und angemessene Leistung bei erhöhten Temperaturen) für fortschrittliche Materialprodukte verwendet. Aufgrund der hochgradig vernetzten Struktur kann es jedoch leicht beim Aufprall versagen [89]. Durch die Zugabe der funktionalisierten Nanocellulose als Verstärkungsmaterial wurden die mechanischen Eigenschaften von Epoxy-basierten Cellulose-Nanokompositen deutlich verbessert [90, 91]. Es wurde ein Ansatz zur Herstellung von Epoxy-Cellulose-Nanofaser-Kompositen mit einer orientierten Struktur durchgeführt [92]. Das Verfahren kombinierte das Ice-Templating-(oder Freeze-Casting-)Verfahren, um hochporöse Nanocellulose-Netzwerke herzustellen, bevor sie als Vorformlinge für die Imprägnierung mit einem Bioepoxidharz verwendet werden. Die Ergebnisse zeigten, dass die Elastizitäts- und Speichermoduli der Nanokomposite in beiden Testrichtungen besser waren als die von reinem Epoxid und die Festigkeit in Längsrichtung verbessert war. Ein weiteres am häufigsten verwendetes duroplastisches Harz ist ungesättigter Polyester (UP). Nanostrukturierte UP-Biokomposite mit einem Nanocellulosegehalt von bis zu 45 Vol.-%, viel höher als in allen früheren Studien, wurden erfolgreich verarbeitet und charakterisiert [93]. Die nanostrukturierte Nanocellulose-Netzwerkverstärkung verbessert nicht nur den Modul und die Festigkeit von UP, sondern auch die Duktilität und Zähigkeit stark. Abbildung 2 zeigt die Bruchmorphologien des hydrophoben Polyesters Poly(3-hydroxybutyrat-co-4-hydroxybutyrat) (PHB), das entweder mit 15 Gew.-% NCC oder 15 Gew.-% acetyliertem NCC unter Verwendung von Feldemissions-Rasterelektronenmikroskopie (FE- SEM). Die FE-REM-Bilder zeigten deutlich, dass acetyliertes NCC im Vergleich zu NCC homogen im PHB dispergiert war. Die Homogenität hat zu der starken Grenzflächenwechselwirkung zwischen dem Verstärkungsmaterial und der Polymermatrix beigetragen [94].
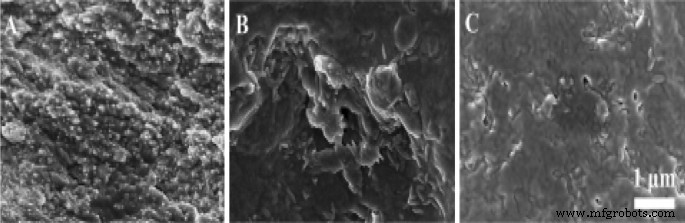
FE-REM-Bilder für die Frakturmorphologien von a PHB/NCC-15, b PHB/acetyliertes NCC(II)-15, c PHB/acetylierte NCC(IV)-15-Nanokomposite. Quelle :Ganet al. [94]. Reproduziert mit Genehmigung von ACS
Tabelle 2 veranschaulicht den Einbau von Nanocellulose in thermoplastische Polymere wie PLA, PVA, Stärke, PU, PP usw. Die zur Entwicklung dieser Nanokomposite verwendeten Verfahren umfassen unter anderem Lösungsmittelaustausch, wässrige Dispersion, Lösungsgießen, Pfropfen, Kern-Rücken-Schaum-Spritzgießen, Elektrospinnen, Koagulation und Thermokompression und anionische Ringöffnungs-Polymerisationsreaktion in situ. Die meisten Studien berichteten, dass die Zugabe von Nanocellulose in Nanokomposite zu einer Verbesserung der mechanischen Eigenschaften (Festigkeit, Steifigkeit, Kriechbeständigkeit, Elastizität), der thermischen Stabilität, der Barriereeigenschaften und sogar einiger Hinweise auf antibakterielle und antioxidative Funktionen der Nanokomposite, abhängig von den Komponenten, führte der verwendeten Nanokomposite. Die Gesamtergebnisse zeigten positive Ergebnisse zur Verstärkung von Nanocellulose in thermoplastischer Matrix, die weitere Studien zur Herstellung umweltfreundlicherer und nachhaltiger Verbundmaterialien anregen und ermutigen.
Natürliche Polymere kommen normalerweise in der Natur vor und können extrahiert werden. Natürliche Polymere sind bis auf wenige Ausnahmen nicht thermoplastisch. Chemische und physikalische Modifikationstechniken sind jedoch in der Lage, die Thermoplastizität in natürlichen Polymeren aus Biomasseressourcen wie Cellulose, Lignin und Chitin auszulösen. Aufgrund des zunehmenden Umweltbewusstseins und der Nachfrage nach grünen Produkten wurden verschiedene natürliche Polymere, die mit Nanocellulose verstärkt sind, verwendet, um Bio-Nanokomposite herzustellen. Es gibt nur eine sehr begrenzte Anzahl von Studien, die über die Verwendung von pflanzlicher Nanocellulose bei der Regeneration von Knochengewebe berichten. Eine der Studien [113] berichtete über die Herstellung eines Nanokomposits durch Synthese von (TEMPO)-oxidierter nanofibrillierter Cellulose (TNFC) oder nanokristalliner Cellulose (NCC) mit Hydroxyapatit (HA). Es wurde festgestellt, dass die Verbundwerkstoffe bessere Druckfestigkeiten, Elastizitätsmodule und Bruchzähigkeit im Bereich des äußeren und dichten kortikalen Knochens aufweisen als die NCC-basierten. Darüber hinaus induzierten die Verbundstoffe keine Zytotoxizität gegenüber menschlichen Knochen-abgeleiteten Osteoblastenzellen, sondern verbesserten vielmehr deren Lebensfähigkeit, was sie vielversprechend für die Knochengeweberegeneration bei lasttragenden Anwendungen macht.
Unter anderen verwendeten natürlichen Biopolymeren sind Natriumalginat, Cellulose und Proteine, die die charakteristischen Eigenschaften wie biologisch abbaubar, biokompatibel und geringe Toxizität aufweisen. Natriumalginat zum Beispiel wird in vielen Bereichen wie Tissue Engineering, Drug Delivery, Lebensmittelverpackung und biomedizinischen Anwendungen in großem Umfang als hervorragendes Biomaterial verwendet. Die geringe mechanische Festigkeit und die unkontrollierten Abbaueigenschaften schränken jedoch seine Anwendung ein. Es wurden mehrere Versuche unternommen, diese Probleme durch die Entwicklung von Nanokompositfilmen mit dem Einbau von Nanocellulose in die Alginatmatrix zu überwinden. Es wurde festgestellt, dass der Einbau von NFC in die Alginatmatrix deren Wasserbeständigkeit und mechanischen Eigenschaften verbessert. Weitere Untersuchungen haben gezeigt, dass TEMPO-vermitteltes oxidiertes NCC mit Ultraschallbehandlung zur Erleichterung der Dispergierung eine bessere Wirksamkeit bei der Verstärkung von Alginat-Biopolymeren im Vergleich zu NFC aufweist. Ein ähnliches Konzept einer bioinspirierten synergistischen Verstärkungsstrategie bei der Integration von Nanocellulose in Chitin, Chitosan, Sojaproteinisolat (SPI) und Leinsamen-Gummimatrix eröffnet einen neuen Weg für die Konstruktion von Hochleistungs-Nanokompositen.
Nanopartikel zur Verbesserung der Eigenschaften von Holzwerkstoffplatten
Holzverbundwerkstoffe werden allgemein als eine breite Palette von Produkten beschrieben, die eine Kombination von Holzelementen aufweisen, die durch ein Bindemittel zusammengehalten werden. Zu den Vorteilen von Holzverbundwerkstoffen gehört, dass sie für bestimmte Qualitäten oder Leistungsanforderungen in verschiedenen Dicken, Qualitäten und Größen ausgelegt werden können. Holzverbundwerkstoffe werden hergestellt, um die natürlichen Festigkeitseigenschaften von Holz zu nutzen (und führen manchmal zu einer größeren strukturellen Festigkeit und Stabilität als normales Holz). On the other hand, wood composites also have disadvantages such that they require more primary energy to manufacture when compared to solid lumber. Hence, wood composites are not suitable for outdoor use as they can absorb water and are more prone to humidity-induced warping than solid woods. The adhesives used release toxic formaldehyde in the finished product. Nanotechnology can be utilised to improve the quality of wood-based composites to fulfil the increasing demand for existing products and for new products to be used in new applications.
The main drawbacks of wood are its susceptibility and biodegradability by microorganisms and also dimensional instability when subjected to varied moisture content. These are mostly due to the cell wall main polymers and their high abundance of hydroxyl groups (OH) [114]. Wood is naturally hygroscopic, and moisture absorption by wood materials is directly related to the exposed surface area. The addition of inorganic nanoparticles to wood composites has been reported to enhance the composites’ anti-microbial properties. Nanoparticles of zinc oxide (ZnO) exhibit good antimicrobial activity. These nanoparticles are added into melamine-urea formaldehyde (MUF) glue before being used for particleboard production [115]. The findings show that there were increments in bioresistance of the particleboards against the Gram-positive bacterium Staphylococcus aureus , the Gram-negative bacterium Escherichia coli , the molds Aspergillus niger and Penicillium brevicompactum as well as the brown-rot fungus Coniophora puteana . Silver nanoparticles which are well-known biocide additives also exhibited similar antibacterial and anti-mold efficiency effects when applied onto the melamine-laminated surfaces of particleboards [116]. The combination of nanocopper oxide and alkane surfactant was also confirmed to improve water and termite resistances of treated plywood specimens [117]. Modified starch-based adhesive was explored as another option to increase the decay resistance of particleboard. Particleboard bonded with modified PVA/oil palm starch added with nanosilicon oxide (SiO2 ) and boric acid was found to be more decay resistant than particleboard bonded with their native starch [118]. The addition of nano-SiO2 and boric acid as the water repellent and antifungal agents, respectively, have prevented the microorganism's activity in the final particleboard.
The manufacture of wood composite panels can be improvised by developing methods to shorten the cure time of the resin during hot-pressing, which could speed up production or improve overall quality of the board. Heat transfer which effects the pressing time of a wood-composite panel varies with thickness, press temperature, closing rate, and mat moisture distribution. The addition of ZnO nanoparticles increased the heat transfer at the centre of the particleboard during hot-pressing causing a greater degree of resin cure and improved the physico-mechanical properties [119]. High conductive nanoparticles such as multiwalled carbon nanotubes (CNTS) and aluminium oxide (Al2 O3 ) were also proven to enhance thermal and mechanical properties of medium density fibreboard [120]. The study also reported that although activated carbon nanoparticles did not give any significant effect to physical and mechanical properties of the board, they have more accelerated effect on the curing of urea formaldehyde (UF) and reduction in the formaldehyde emission compared to the other two nanofillers.
In fabricating the wood composites, the adhesives play exceptionally significant role which affect the composites properties which include the mechanical properties, their ability to perform in wet conditions and their effects on the environment. Urea formaldehyde, melamine urea formaldehyde and phenol formaldehyde are commonly used in the wood composites industry. The utilisation of nanoparticles has led to improvements of the properties of adhesives. Many studies have been conducted to produce nanomaterial-reinforced wood composites with enhanced physical and mechanical performance and reduced formaldehyde emission. Nanoclays have been shown to be excellent fillers and reinforcement for the resin matrix, and significantly enhancing strength, toughness and other properties. The modification of UF adhesive using nanoclay particles for plywood fabricated with three forest species from fast-growth plantations:Cordia alliodora , Gmelina arborea and Vochysia ferruginea were reported by Muñoz and Moya [121]. It was determined that nanomodification of the resin with nanoclay at 0.75% improved the moduli of rupture and elasticity of the board. The effect of using nanoclay particles in PF adhesives significantly elevated mechanical properties of the adhesive in the bondline and contributed an increase in the macro-bonding strength of plywood [122]. Interestingly, transition metal ion-modified bentonite (TMI-BNT) nanoclay was used to covert crystalline UF resins to amorphous polymers by blocking the hydrogen bonds via in situ intercalation method [123]. This resulted in 56.4% increase in the adhesion strength and 48.3% reduction in the formaldehyde emission.
Other nanoparticles used to improvise the physical and mechanical properties of wood composites include nanowollastonite (NW) [124, 125] nano-ZnO [126], nano-SiO2 , nano-Al2 O3 [127], nanosilver [128] and nanocellulose (NCC and NFC). The NCC was utilised as filler for the adhesive, whereas NFC was applied as a binder to the formulation of the composite boards. Recently, it has been revealed that the addition of micro- and nanofibres of cellulose have advantageous effects on the properties of resin. Based on the investigations, it was found that the addition NCC significantly improved the mechanical properties of plywood in the amount of 10%/100 g of solid resin [129]. On the other hand, the physical properties of particleboard after adding the NCC to UF adhesives showed smooth surface, insignificant difference in the density and moisture content of the panels and only high value of nanocellulose content exhibited significantly higher in thickness swelling [130]. Meanwhile, particle boards panels manufactured using NFC as the bonding materials were shown to meet the industry requirements in terms of mechanical properties for low density grades [131]. For high density particleboard, it was estimated that the increased NFC ratio and higher pressure could improve internal bond properties. Even the nail and face screw withdrawal strength was found to be increased with the increase in NFC addition ratio and panel density [132, 133].
Wood Coating
Forests are primarily or partially used for the production of wood and non-wood forest products. Non-wood forest products include bamboo, rattan, firewood, charcoal, damar, palm, etc. There is a huge demand for high quality wood, but the availability of wood from natural forest has been declining. Consequently, the search for non-wood resources as an alternative to wood has been accelerated. Due to its rapid growth property, bamboo has been developed into one of the most important non-wood forest products. Wood is a natural biologically self-assembled polymeric structure (cellulose, lignin, hemicellulose). It is one of the most versatile materials and has been used for centuries in the form of building and structures. However, wood is subjected to intense oxidative degradation processes such as photo-oxidation, chemical oxidation, thermal decomposition and photolysis reactions from the environment, including ultraviolet (UV) light, moisture, chemical pollutant and heat/cold variations [134]. Even non-wood materials like bamboo itself is a natural organic material which is rich in protein, carbohydrate and other nutrients and is prone to mildew, being eaten by moths and rotting. Hence, the final products of wood and non-wood products conventionally comprise additives which can be used as coatings for protection and aesthetic appearance improvement, preservatives for protection against fire and biological factors (fungi and insects).
Coating is a process of applying a layer to the substrate surface. Examples of common coatings applied to wood surfaces are varnishes, lacquers, and paints whose purpose can be both protective and decorative. The main components of the coatings determine their fundamental properties such as binders, pigments, solvents, fillers, and additives [135]. Each element contributes specific properties to the wood surface. The binder contributes to the adhesion of the pigment to the wood and creates a protective layer, while the pigment provides colour and form non-translucent surface layer. The solvents give necessary viscosity for coating application, and the addition of the fillers alters the colour strength and the gloss of the coating. As for the additives, they inhibit mould and decay, assist the drying process, improve the adhesion properties and control the finishing. However, there are weaknesses of coatings such as limited flexibility, strength loss, disproportionate adhesion between coating layer and substrate, inferior abrasion resistance and less durability.
Nanocoating has the capability to resolve these issues. Nanocoating is a process by which a thin layer of thickness about < 100 nm is deposited on the substrate for improving some properties or for imparting new functionality. The nanocoating can be used not only on nanomaterials but also on a bulkier material with an extremely thin layer coating without affecting the topography of the substrate surface. The application of nanocoating in wood and wood products is mainly focused on the improvement of durability, mechanical properties, fire resistance and UV absorption as well as decrease in water absorption. One approach to enhance the functionality and the end user value of nanocoating is the addition of nanoparticles [136]. These nanoparticles have very large surface-to-volume ratios due to their morphology, which allows them to interact intensively with their surroundings, and their nanosize ensures transparency is still sustained.
Nanoadditive for Durability Improvement
Nanocoatings are able to improve the durability of wood and non-wood products by utilising nanoparticles and nanodelivery systems that make the changes at the molecular level of the products. One of the aims of coatings is to prevent the growth of various microorganisms like fungi and bacteria. Nanosized particles of metal oxides, such as zinc oxide (ZnO) [137, 138], titanium oxide [138, 139] and cerium oxide (CeO2 ) [140] were reported to demonstrate strong antimicrobial properties. Studies have been conducted in the direct deposition of nanoparticles onto wood surfaces or direct functionalisation of wood surfaces with nanoparticles. ZnO nanoparticles were successfully fabricated on the surface of bamboo timber by a simple low-temperature wet chemical method based on sol–gel-prepared ZnO seed layers. The findings indicated that the treated bamboo timber had better resistance against Aspergillus niger V. Tiegh (A. niger) and Penicillium citrinum Thom (P. citrinum), but poor resistance against Trichoderma viride Pers. ex Fr (T. viride) [141]. Graphene also demonstrates superior ability to inhibit bacterial growth. Hence, the combination of utilising reduced graphene oxide and nano-ZnO to coat bamboo-based outdoor materials via a two-step dip-dry and hydrothermal process, resulting in the improvement of the mould resistance and antibacterial activity properties [142]. Similarly, the nanostructured ZnO using a hydrothermal process has also provided an effective protection of wood surfaces from biodeterioration [143].
Waterborne polyurethane [144] coatings (WPU) incorporated with nanocrystalline cellulose (NCC) and silver nanoparticles (AgNPs) were used to improve antibacterial property of wood board [145]. The AgNPs were known for their antimicrobial material but aggregated easily during the preparation process. Therefore, NCC was introduced to assist with the blending and dispersibility of AgNPs with WPU or other coatings. In addition, NCC was also a good reinforcing agent to improve the mechanical properties of nanocomposites. Mini emulsion polymerisation was also used to synthesise an acrylic latex coating containing AgNPs, which will limit the growth of black-stain fungi on the wood surfaces [146]. The study of the antibacterial effect of silver and zinc oxide nanoparticles in acrylic coatings applied during the treatment of commercial wooden composites such as particleboard and medium density fibreboard was conducted [147]. Ag and ZnO nanoparticles were partly more effective against the Gram-negative bacterium Escherichia coli compared to the Gram-positive bacterium Staphylococcus aureus .
Nanoadditive for Water Absorption Improvement
It is well known that wood is susceptible to water or moisture. This is due to the hydrophilic nature of the cell wall constituent polymer and its capillary-porous structure. The interaction between wood and water leads to biodegradation of wood, dimensional instability and accelerated weathering. Although there are conventional chemical modifications being used to improve the hydrophobicity of wood, the accessibility of water into wood is still not completely retarded [148]. Furthermore, the chemicals used in the treatment process are possibly hazardous. Hence, nanotechnology is used as an alternative approach for wood modification and functionalisation. The incorporation of nanoparticles into polymeric coatings is used to improve water absorption of wood surfaces. Two approaches are utilised to integrate the nanoparticles into the coatings, namely solution blending and in situ addition [149]. The first approach (solution blending) is when a solvent combines with the polymer before being dispersed onto the wood surfaces. This physical method can be applied through dipping, brushing and spraying [150]. Wuet al. [151] reported that a superhydrophobic coating was constructed on the surface of poplar wood with a contact angle of up to 158.4° through the waterborne UV lacquer product (WUV) which was modified by ZnO nanoparticles and stearic acid. Compared with WUV, the water resistance of zinc stearate/waterborne UV lacquer super-hydrophobic coating (ZnSt2 /WUV) was stronger, which was conducive to prepare superhydrophobic coatings in an easy and environmentally friendly. Interestingly, a water-based varnish added with TiO2 nanoparticles was used to evaluate the finishing of nine tropical wood species [152]. It was found that the incorporation of TiO2 nanoparticles decreased the values of water absorption and after a year of weathering exposure, the varnish with no added TiO2 nanoparticles degraded completely, while the modified varnish film endured. Other examples of superhydrophobic wood coatings which were successfully prepared are lignin-coated nanocrystalline cellulose (L-NCC) particles/polyvinyl alcohol (PVA) composite paint system [153], UV-light curable methacrylic-siloxane-cellulose composite coatings [154], Fe 3+ -doped SiO2 /TiO2 composite film [155] and polydimethylsiloxane (PDMS)/silica hybrid coating system [156].
The second approach is the in situ addition or a chemical process which involves compound addition directly to monomers and subsequent polymerisation. The nanoparticles are synthesised in situ by chemical reactions on the wood surface such as hydrothermal methods or solgel deposition. Gao et al. [157] applied a simple and effective method in preparing superhydrophobic conductive wood surface with super oil repellency using AgNPs modified by fluoroalkyl silane. The multifunctional coating could be commercialised for various applications, especially for self-cleaning and biomedical electronic devices. In another study, bamboo was treated using ZnO sol, and the ZnO nanosheet networks were grown hydrothermally onto the bamboo surface and subsequently modified with fluoroalkyl silane [158]. The successfully treated bamboo exhibited superior properties such as robust superhydrophobicity, stable repellency towards simulated acid rain, UV-resistant and fire-resistant. Similar superior properties were obtained when bamboo timber prepared by the hydrothermal deposition of anatase TiO2 nanoparticles and further modified with octadecyltrichlorosilane [159]. Superhydrophobic wood surfaces can also be prepared using approaches such as layer-by-layer [160] assembly of polyelectrolyte/TiO2 nanoparticles multi-layers and hydrophobic modified with perfluoroalkyltriethoxysilane (POTS) [161], spray coating of a waterborne perfluoroalkyl methacrylic copolymer (PMC)/TiO2 nanocomposites onto the PDMS pre-coated substrate [162] and a one-step hydrothermal process using tetrabutyltitanate (Ti(OC4 H9 )4 , TBOT) and vinyltriethoxysilane (CH2 CHSi(OC2 H5 )3 , VTES) as a co-precursor [163]. Even a biomimetic approach as to produce a lotus-leaf-like SiO2 superhydrophobic bamboo surface based on soft lithography was successfully carried out [122].
Nanoadditive for Mechanical Properties Improvement
The inorganic particles integrated into organic polymers are commonly used in wood coatings to increase the mechanical properties. As fillers, the rigidity and hardness of the inorganic materials are combined effectively with the polymer’s processability. The inorganic particles when apply in micron size have disadvantages such as they reduce the flexibility of the material and decrease the transparency of the coating system [164]. The utilisation of the inorganic particles in nanosize increases the surface area and the ratio of the interfacial area, which subsequently influences the properties of the raw material [165]. Recent studies have investigated on using nanocellulose as a renewable reinforcement to develop a bio-based nanocomposite coating system with improved performance. Nanocellulose was surface modified due to the issue of incompatibility with the polymer matrix. The addition of TEMPO-oxidised cellulose nanofibres improved the mechanical properties of the WPU coating [144, 166]. In the case of the non-polar polymer matrix [167], nanocrystalline cellulose was modified by two methods, with acryloyl chloride or a cationic surfactant [167]. An increase in NCC loading level up to 2% increased hardness, elastic modulus, and tensile strength.
Nanosilica is another common nanoparticle that is applied for the improvement of mechanical properties. Among the advantages of using nanosilica are its high hardness and can easily be chemically modified to improve its compatibility with the polymer matrix. A recent study by Meng et al. [168] reported castor-oil-based waterborne acrylate (CWA)/SiO2 hybrid coatings with organic–inorganic covalent cross-linked network structures were prepared via solgel and thiol-ene reactions. The finding showed that beside the emulsions had good stability, the thermal and mechanical properties of the coating improved significantly at 10 wt% of SiO2 . The improvement of mechanical properties with nanosilica addition was also described in other coating system such as waterborne nitrocellulose [169] and acrylate [170].
Nanoadditive for UV Absorption Improvement
The process of wood photodegradation begins directly after being exposed to solar light, and then the colour changes and progressive erosion of the wood surface occur. The UV radiation is capable of photochemically degrading the polymer structure components of wood (lignin, cellulose and hemicellulose) [171]. The photodegradation process usually results in reduced water resistance of wood and wood-based materials which lead to further biodegradation under outdoor exposure conditions. The intense damage to materials due to the UV component in solar radiation can be prevented by using light-stabilisation technologies, surface coatings or by replacing these materials with materials that are more resistant against UV radiation [172]. Nanoparticles can be utilised to improve the UV resistance for solvent, waterborne and UV coatings in order to protect the wood surfaces. Nanoparticles that contain functional coatings to achieve UV-blocking properties offer a high level of protection against UV without affecting the transparency of the surface. The small size of the nanoparticles gives a significant increase in effectiveness of blocking UV light compared to natural material due to their large surface area-to-volume ratio.
The use of UV radiation absorptive coatings serves to prevent lignin degradation from UV light. Among the nanoparticles used as UV absorbers are mainly TiO2 and ZnO. Wallenhorst et al. [173] reported a system composed of a Zn/ZnO coating and additional polyurethane sealing strongly reduced photodiscolouration of the wood surface and proved to be chemically stable. The combination of benzotriazole (BTZ) and ZnO nanoparticles was applied as the UV absorbers in acrylic-based bamboo exterior coatings [174]. Strong synergistic effects were detected in the BTZ–ZnO coatings, especially for the 2:1 ratio formulation. The coating system provided high resistance to photodegradation and effectively inhibited photodiscolouration of the bamboo substrates. Another mixture of benzotriazoles, hindered amine light stabilizers (HALS), and ZnO nanoparticles in thick-film waterborne acrylic coating also gave the most positive effect in UV protective surface modification when applied to oak wood [175]. The mixture of benzotriazoles, HALS and both TiO2 and ZnO nanoparticles was confirmed as one of the most effective treatments for colour stabilisation of wood due to UV and VIS spectrums. It was reported that wood specimens coated with rutile TiO2 and a mixture of methyltrimethoxysilane and hexadecyltrimethoxysilane showed superior weathering performance and improved resistance to surface colour change and weight loss [176]. Das TiO2 coating also was found to apparently enhance the colour stability of wood during UV light irradiation without water spray. However, the adjacent wood surface degraded because of the photocatalytic activity of TiO2 [177].
Nanoadditive for Fire Retardancy Improvement
The flammability of wood and non-wood products has restricted utilisation, with fire safety being a major concern for the various applications. To overcome the inherent deficiencies and use of wood and non-wood in a safe manner, the flame retardant properties need serious consideration. Nanoparticles have recently been used to produce the nanocomposites for the improvement in fire retardant properties. The utilisation of nanoparticles, either alone or in combination with conventional fire retardants, serves to reduce the ignitability of wood. The nanosize and high surface area of nanomaterials make them more effective at low concentrations than other conventional compounds which are an enormous advantage industrially and economically. The surface modification is necessary for nanoparticles to achieve better compatible and homogeneous dispersion. Das TiO2 coated wood was found to be capable in reducing the flammability of the wood and the spreading of the flame, as compared to the uncoated sample [178]. The ZnO–TiO2 -layered double-nanostructures had been synthesised on a bamboo substrate [179]. The findings showed that the oxygen index increased from 25.6 to 30.2% after being covered with a ZnO–TiO2 coating, which revealed a significant enhancement of its flame retardant property. Layered double hydroxides [180] can absorb a large amount of heat, dilute the concentration of flammable gas, and absorb harmful acid gases during the decomposition process; therefore, it is an excellent flame retardant. Yao et al. [180] applied nanomagnesium aluminium layered double hydroxide (Mg–Al LDH) to bamboo in an in situ one-step process and found that the total heat release and total smoke production were reduced by 33.3% and 88.9%, respectively, compared to those of samples without Mg–Al LDH. Wanget al. [181] introduced zinc-aluminum layered double hydroxide (Zn–Al LDH) nanostructures to wood and found that the peak heat release rate (PHRR) and total smoke production were reduced by 55% and 47%, respectively, compared to those of the pristine wood [181]. Nanostructured carbon materials such as graphene was also proven to have a great potential to be used as an effective fire retardant in wood and wood-composite materials for surface protection against fire [182].
Wood Durability
Wood is such a versatile material that finds its use in various fields like construction, furniture and artwork [183,184,185]. Wood is applied as a construction material due to its high strength-to-weight ratio, eco-friendly characteristic, aesthetic appearance and biodegradability feature. Unfortunately, wood is very sensitive to biological attacks, especially by decay fungi and insects [186, 187]. Wood also gets affected by exposure to UV-radiation, fire and moisture [188]. Moisture can cause wood warping, cracking and dimensional instability. The wood degradation can cause aesthetical and internal structural damages. The degradation of wood leads to immeasurable losses each year. Besides, the growth of decay fungi on wood structural will trigger health problem to human including allergies, respiratory symptoms and asthma especially after prolonged indoor exposures [189]. Therefore, the associated issues related to wood cannot be simply overlooked. Preservation of wood using chemical is the effective way to protect and prolong the service life of wood.
Over the last decades, many chemical preservatives have been developed and used to protect wood against biodegradation agents [188, 190]. Unfortunately, most of these chemical preservatives may pose serious effects to human, living organisms and environment due to their accumulation in soil and ecosystems [191]. Chromated copper arsenate (CCA) is one of the chemical preservatives widely used since the middle 1930s and effectively protects wood against decaying fungi, termite and insect borer. However, CCA was shown to be toxic to human and environment [192,193,194]. A similar issue is also faced by another chemical, i.e. pentachlorophenol (PCP). It was considered to be hazardous to human health; thus, its production and uses were banned in many countries [195].
In response to these issues, a new series of chemical preservatives claimed as safe and less-polluting are being introduced. These chemical preservatives can be obtained from plant extract or produced synthetically and have plant bioactive compound properties. For instance, pyrethroids, derived from pyrethrum flower (Chrysanthemum cinerariifolium ), have a potent insecticidal activity and can now be produced synthetically. Unfortunately, these chemicals were found easily degradable by light and temperature, and have narrow efficiency spectrum [196]. Other limitation of organic pesticides is that most of them dissolve in organic solvents and cannot be formulated as water-based wood preservatives. Therefore, smart and intelligent organic pesticides delivery system is crucial. Through a smart delivery system, biocides can be delivered in a controlled and targeted manner. This will reduce hazards to human and environment [17].
Wood treatment via nanotechnology method can improve wood durability against biodegradation agents and weather [14, 197, 198]. The main advantage of applying nanoproducts to wood is its unique ability to penetrate deeply in wood structure [14, 199], thus improving durability properties that result in a long life time service. On the other hand, complete penetration with uniform distribution of nanoproducts can also be achieved [200]. To date, many ready-to-use nanoproducts (nanopreservatives) for wood protection are available in the market, while some of them are still in the research and development stage. Generally, nanoproducts used to protect wood can be classified into two types, namely nanocapsules and nanomaterials. Nanocapsule refers to the pesticides embedded in a polymeric nanocarrier, while nanomaterial is nanosized metals which can be directly impregnated into wood [201].
Biocide Enhancement Property via Nanoencapsulation
Encapsulation of pesticides into polymeric nanocarriers is one of the promising nanotechnology techniques in improving the impregnation of wood with pesticides. This technique is designed to increase the solubility of poorly water-soluble pesticides and to release pesticides in a slow manner [202]. Encapsulation allows those low solubility pesticides to disperse easily in solid polymeric nanoparticles. The polymer can then be suspended in water and applied to wood via conventional water-based treatments [149]. Encapsulation is also able to protect the hydrophilic active ingredient from excessive leaching [19]. Due to the small diameter of capsule, it can be easily incorporated and penetrated into the cell wall of the wood. This accordingly improves the durability of treated wood against biodegradation agents. Not only this technology can deliver pesticides safely, it can also prolong the pesticide lifespan, resulting in extended protection for the wood [203].
Encapsulation of pesticides is a bottom-up approach. It can be carried out through several techniques such as nanoprecipitation [17, 204,205,206], emulsion-diffusion [207] and double emulsification [208] (Table 3). The materials used as polymeric nanocarriers can be derived from natural polymer, synthetic polymer or combination of both [209]. For instance, natural polymer includes cellulose, starch, alginates, silica and halloysite. The synthetic polymer usually used as polymeric nanocarriers includes poly(vinyl acetate), poly(methyl methacrylate), poly(lactic acid), etc. (Table 3).
A previous study by Liu et al. [18, 216] successfully encapsulated tebuconazole and chlorothalonil into polyvinylpyridine and polyvinylpyridine-co-styrene using the impregnation method. The particle size of capsules obtained was between 100 and 250 nm. They impregnated a suspension of the capsules into sapwood of southern yellow pine and birch using conventional pressure treatments. The treated wood was then exposed to brown-rot (Gloeophyllum trabeum ) and white-rot (Trametes versicolour ) fungi for 55 days. The results showed great resistance against both decay fungi.
Salma et al. [17] used the nanoprecipitation technique to encapsulate tebuconazole. The polymer capsules containing tebuconazole were prepared from amphiphilic copolymers of gelatine grafted with methyl methacrylate with the size diameters ranging from 200 to 400 nm or 10 to 100 nm depending on core/polymer shell mass ratio. The encapsulated tebuconazole was reported to be able to preserve wood against a brown rot fungus. On the other hand, the formulation system developed by Salma et al. [17] is flexible and can be easily modified using copolymerisation of other acrylic monomers like hydroxyethyl methacrylate. This indicates that tebuconazole release rate can be tailor-made. However, the disadvantage of this nanocapsules is that they are prone to aggregation, which reduces the delivery efficiency of the nanocapsules into the wood.
Can et al. [217] successfully encapsulated nano silver into polystyrene-soybean co-polymer. In their study, the Scots pine was impregnated with the capsules and tested against white-rot fungi (Trametes versicolor ). The finding obtained from the study indicates that the soybean oil, polystyrene and nanosilver played important roles in the synergistic effect of increasing the decay resistance of Scots pine.
Impregnation of Metallic Nanoparticles for Durability Enhancement
Metallic nanoparticles have been used to protect wood against biodegradation agents and weathering since decades ago [197, 218, 219]. Nanoparticles offer better characteristics than their bulk form, mainly because of their reduced size that leads to high specific surface area-to-volume ratio, uniform size distribution and good stability. Due to its very small size, nanoparticles can penetrate deeply and uniformly into the wood pores leading to a protection of wood [197, 220]. In addition, dispersion stability is improved because of the size and also by addition of surfactant [221]. Dispersion stability coupled with small particle size may greatly improve the following aspects:(1) preservative penetration, (2) treatability of wood, (3) stability of finishes and coating products, (4) low viscosity, and (5) non-leachability [222, 223]. Besides, it can enhance compatibility with binders thereby being able to increase the affinity with wood polymers [197, 224].
Metallic nanoparticle can be prepared by altering the particulate size of metal via chemical reactions, mechanical treatment, heating or refluxing. To date, a lot of nanoparticles have been used for wood protection. Metallic nanoparticles mainly copper, silver, boron and zinc exhibited a good performance against white-rot and brown-rot but less efficient against mould [219]. Titandioxid (TiO2 .) ) is another nanoparticle that has a good potential to be used as wood preservative. The potential of TiO2 is related to its antibacterial and antifungal [14, 225] and UV-resistant properties [225, 226]. However, the study on wood treated with TiO2 is still under preliminary investigation [14, 225]. Table 4 lists the works on the utilisation of TiO2 for wood preservation. The distribution of TiO2 in wood was studied by Mohammadnia-Afrouzi et al. (Fig. 3).
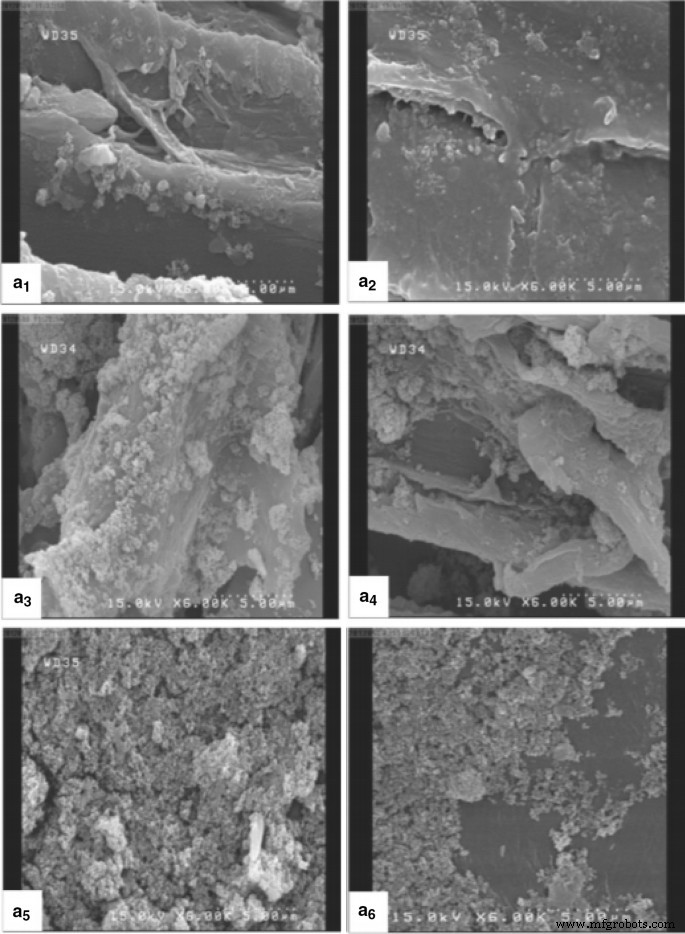
SEM images taken from the tangential section of nano-TiO2 wood treated samples:the 0% moisture content (MC)/0.5% concentration (a1), the 25% MC/0.5% concentration (A2), the 0% MC/1% concentration (A3), the 25% MC/1% concentration (A4), the 0% MC/1.5% concentration (A5) and (25% MC/1.5% concentration. Source :Afrouzi et al. [227]
Copper is an essential biocide for wood protection. However, copper alone fails to protect wood against copper-tolerant wood destroying fungi. Copper nanoparticles is a new generation of wood preservative-based copper. The use of copper nanoparticles instead of conventional copper shows improved durability of wood against decay fungi [230]. This shows that copper nanoparticles can be used to protect wood without the presence of chromium and arsenic [230].
Cristea et al. [15] studied the effects of the addition of ZnO nanoparticles and silver nanoparticles into exterior wood coatings. The purpose of the study is to improve the durability and wood protection through UV shielding. Besides providing an efficient protection against UV, the mechanical properties of wood such as hardness, adhesion strength, the abrasion resistance and the barrier effect for water vapor diffusion were slightly improved.
The mixture of ZnO nanoparticles with silver nanoparticles was able to protect wood from weathering problem such as UV rays [231]. They impregnated sapwood of cottonwood using three different concentrations of mixture by full-cell process. The samples were then exposed to natural weathering. The colour changes of treated wood samples were measured using spectrophotometer. The wood treated with ZnO nanoparticles alone was used as a control. The finding indicates that wood treated with the mixture of ZnO nanoparticle and silver nanoparticles has the lowest colour changes compared to the samples treated with each metallic nanoparticles.
In another study, Mantanis et al. [197] treated black pine wood with ZnO, zinc borate and copper oxide nanoparticles under vacuum. They used acrylic emulsion to force the metallic nanoparticles into the wood structure to avoid leaching. The durability of treated wood against mould decay fungi and subterranean termites was evaluated. Results showed that wood treated with zinc borate slightly inhibited the mold, while the other metallic nanoparticles did not exhibit mould. A similar finding was reported by Terzi et al. [232] that ZnO nanoparticles did not exhibit the mould growth on wood specimens. However, all metallic nanoparticles significantly inhibited white-rot fungi and termite.
Another study shows that metallic nanoparticles are able to improve fire retardant properties of wood. Francĕs et al. [16] studied the effect of SiO2 , TiO2 and ZnO2 infiltrated into pine veneers. They reported that veneer treated using 3 wt% of SiO2 was most effective to improve the fire retardant behaviour.
Despite the remarkable advantages of nanotechnology in the wood preservation sector as discussed above, the fundamental understanding on synthesis, processing and characterisation of nanocapsules and metallic nanoparticles for wood protection still needs to be improved. The most interesting characteristics need to be considered during the design and development of nanocapsules or metallic nanoparticles for wood protection are as listed in Table 5.
Potential of Nanocellulose-Based Material in Energy Sector
Energy is an important resource that has a strong correlation between economic growth and development [233]. Today, the main energy sources are from fossil fuels and hydroelectric sources, which are very harmful to environment because they can cause climate change and global warning as well as ozone layer depletion, pollution, greenhouse gases emission and ecological destruction [234,235,236]. About 80% of carbon dioxide (CO2 ) emissions in the world are from energy sector and technology advancement is required to develop sustainable renewable energy resources to reduce the CO2 emissions as well as to overcome the global warming impact on life and health in line with the needs of accelerating technology development [234, 237].
In order to minimise the environmental effects, a sustainable and low-cost energy efficient carbon-based material has been explored as a potential to replace some conventional materials in the fabrication of energy devices. One of the natural carbon-based materials is cellulose which is the most promising natural polymer with many usages, including energy [237].
Nanotechnology is one of the advanced technologies that have the potential and prospect to fulfil the demand to create clean and green energy. Developing this new material in nanoscale enables new application and its interaction with current energy technology that would revolutionise the energy field from usage to supply, conversion to storage and transmission to distribution [238]. By adapting this nanotechnology, it will have high impact on the development of clean and green energy and benefits the environment and natural resources [239].
According to Serrano et al. [234] and Hut et al. [235], most promising application of nanotechnology for energy conversion is mainly focused on solar energy, conductive materials, solar hydrogen, fuel cells, batteries, power generation and energy devices. Understanding the structural and morphological properties of nanomaterial is essential to obtain the proficiency and sustainability for many applications. The greatest application of nanotechnology in energy generation is solar energy using photovoltaic (PV) cells which focuses on harnessing efficiency [233]. Consumption of energy generated from this solar cell using natural resources will reduce the usage of fossil fuel and decrease the pollution towards creating environmentally friendly and green energy [239]. In addition, the development of nano devices using solar cell could improve the existing materials efficiency as well as reducing manufacturing cost that might increase the economic growth [236].
Nanotechnology has been used in various applications to improve the environment, to solve humanity problems and to produce more efficient and cost-effective energy, such as generating less pollution during products manufacturing, producing solar and fuel cells at a competitive cost, hydrogen production, cleaning up organic chemicals polluting groundwater and overcoming the problem of energy sufficiency, climate change and diseases as well as to reduce the dependency on non-renewable energy sources [233, 235, 240].
Nanocellulose-Based Material for Solar Energy
Solar energy is available in various parts of the world and can be captured from the sun with 15,000 times more energy yearly. This energy source can be used in different ways:photovoltaic (PV) technology, solar thermal systems, artificial photosynthesis, passive solar technologies and biomass technology, which are used to produce electricity, steam or biofuels [234, 235].
In future, nanotechnology might contribute to develop an effective and low-cost system for production, storage, and transporting of energy [235]. According to Serrano et al. [234] and Hut et al. [235], current photovoltaic (PV) market is based on silicon wafer-based solar cells (first generation) and thin film layers of semiconductor materials (second generation). Current drawback of using solar cells is the cost of manufacturing mainly on the high cost of conventional PV cells with poor energy absorption efficiency (less than 40%) [233].
Nanocellulose shows a good potential to be used in the solar energy system due to its renewability, biodegradability, biocompatibility, broad modification capacity, adaptability and versatile morphology [241, 242]. Low cost, flexible and porous substrate of cellulose could be used to produce solar cells. Nanofibrillated cellulose (NFC) with size as low as 4 nm could become the excellent candidate for production of ultrathin paper for use in solar cell component to store the energy [235]. Klochko et al. [243] used nanocellulose from biomass for the development of biodegradable eco-friendly flexible thin film as a thermoelectric material. The thin film was used to convert low-grade waste heat from sun radiation into electricity at near-room temperature.
Nanocellulose-Based Conductive Materials
Conductive materials allow the flow of electrical current which is needed in the fabrication of energy devices. There are many types of conductive materials such as conductive polymers and conductive carbon materials (e.g. carbon nanotubes, graphene, and carbon black) and metallic particle with different levels of conductivity. These conductive materials can be combined with nanocellulose to form novel composites. The process of production conductive nanocellulose is shown in Fig. 2. There are two major strategies involved in nanocellulose based conductive hybrids fabrication process; one is coating of conductive materials layer on the surface of nanocellulose substrates, and another one is mixing the conductive materials inside the nanocellulose substrate to make composite [237]. Conductive polymers are an alternative to metallic materials because of their good electrochemical performance, light in weight and low cost. One of the most promising conductive polymers is polyaniline (PANI) because of its simple route of synthesis, controllable conductivity and high specific capacitance [244].
Nanocellulose-based conductive materials are developed for supercapacitors and energy storage device applications using various types of method such as in situ polymerisation, doping, coating, inkjet printing and in situ depositing [68, 244,245,246]. Modification of the existing supercapacitor by adding nanocomponents has increased its ability to store large amount of energy with longer time of supply [238].
Besides that, nanocellulose-based composite membrane electrodes can be developed via in situ polymerisation of nanocellulose using conductive components via a simple filtration unit (Fig. 3). A well-mixed conductive materials/nanocellulose composite membrane is left on the filter after the liquid has passed through the filter and air-dried composite membrane can be peeled off from the filter membrane for further use as supercapacitors [244].
Nanocellulose for Energy Storage
The potential application of nanocellulose for energy storage application has gained much attention recently. This is due to its nanoscale dimension, high surface area-to-volume ratio, and rich with hydroxyl group, which make their surface chemistry easily modifiable for composite processing. The most important aspect in energy storage is to develop nanocellulose with conductivity and flexibility properties. It can be achieved by adding conducting polymers such as polyaniline and polypyrrole. For example, the nanocellulose/polyaniline composite film is widely used as paper based sensors, flexible electrode, and conducting adhesive [68, 247,248,249]. Razaq et al. [250] manufactured electrodes from the composite of nanocellulose/polypyrrole and carbon filament for paper based energy storage devices. Wanget al. [248] reported that their devices which developed using composite of nanocellulose/polypyrrole provide high charge and discharge rate capabilities, high cell capacitances, and cycling performance.
Nanocellulose-Based Materials for Lithium and Vanadium Battery
High demand on flexible portable electronic devices recently such as smart phone, electrical vehicles, laptops, and even the grid energy storage causes increasing demand on rechargeable lithium-ion battery (LIBs) [251] and supercapacitors [252]. LIBs are one of the most ideal energy storage candidates for electronic devices, due to their high energy density, moderate power density and cycle stability. In LIBs, electrolyte is important for lithium-ion (Li + ) transfer between anode and cathode. Organic liquid electrolyte is used for LIBs system, but it can pose tremendous safety concern due to the high toxicity and flammability. Solid-state electrolyte has become of interest because it demonstrates obvious advantages of low flammability and low toxicity [253, 254]. According to Janek et al. [255], solid-state electrolyte is classified as solid polymer electrolyte (SPE) and inorganic solid electrolyte (ISE). However, SPE offers the advantages of easy processing and flexibility [255].
Qinet al. [256] developed SPE by incorporating polyethylene oxide (PEO) with nanofibrillated aerogel and bis(trifluoromethanesulphonyl)imide lithium salt (LiTFSI). The results showed that the ionic conductivity properties of SPE were significantly enhanced due to the negatively charged nanofibrillated cellulose. The results also proved that the fabricated SPE is electrochemically stable, mechanically robust and thermally stable as well as flexible, expected for use in flexible electronic devices.
Nair el at. [257] fabricated nanocellulose-laden composite polymer electrolyte for high performing lithium-sulphur batteries using a thermally induced polymerisation method. The composite polymer electrolyte demonstrates excellent ionic conductivity, thermal stability up to more than 200 °C and stable interface towards lithium. The electrolyte also has stable cycling profiles which are attributed to significant reduction of the migration of polysulphide towards anode by entrapment of nanocellulose in the polymer matrix.
Another study was carried out by Zhang et al. [258] on robust proton exchange membrane developed using sulphonated poly(ether sulphone) reinforced by core–shell nanocellulose for vanadium redox flow batteries (VRFBs). It was found that with the incorporation of silica–encapsulated nanocellulose, the proton exchange membrane exhibits outstanding mechanical strength of 54.5 MPa and high energy efficiency above 82% at 100 mA cm −2 , which is stable during 200 charge–discharge cycles. Proton exchange membrane is one of the key components in VRFBs. It functions as the separator to avoid vanadium ions crossover. It also acts as proton conductors that contribute to high voltage efficiency in VRFBs [259].
Nanocellulose-Based Materials for Flexible Supercapacitor
Supercapacitor or known as electrochemical double-layer capacitor or ultracapacitor is another versatile energy storage system that has gained the attention of researchers worldwide [260]. With the high power density, long life cycle, simple principles, low maintenance, portability, stable performance and fast charge/discharge rate make supercapacitors capable of filling the gap between batteries and conventional capacitors [260]. These properties offer a promising approach to meet the growing power demands.
Electrode is a very important component in supercapacitor. It requires good electrochemical performance and flexibility especially for preparing a high-performance flexible supercapacitor. Him et al. [261] and Zhe et al. [262] found that graphene and nanocellulose are excellent flexible electrode materials for supercapacitors. Nanocellulose has been used as a substrate material because of its good biodegradability, mechanical strength, flexibility, and chemical reactivity. The porous structure and hydrophilicity of nanocellulose can facilitate the attachment of other materials for example graphene in their fibrous network structure [263]. At the same time, the abundance of hydroxyl groups on the nanocellulose surface enables the interaction of nanocellulose with other polymer to form strong composites [264].
Khosrozadeh et al. [265] developed an electrode for supercapacitor using nanocellulose-based polyaniline/graphene/silver nanowire composite and after applying it for 2400 cycles, at a current density of 1.6 A/g, the supercapacitor showed a power density, energy density and capacitance of 108%, 98% and 84%, respectively. This shows that the electrode has an excellent cyclic stability and good mechanical flexibility. On the other hand, Ma et al. [266] developed an electrode using bacteria cellulose/polypyrrole coated with graphene. The prepared electrode has a good mechanical flexibility in which it can bend at any angle. The area capacitance and energy density can reach 790 mF cm −2 and 0.11 mWh cm −2 , respectively, when assembled into symmetric supercapacitors. The nanocellulose-graphene electrode can be fabricated using chemical cross-linking or physical cross-linking method [267, 268]. Nanocellulose as an electrode component of supercapacitor also plays the role of internal electrolyte reservoir. This is because nanocellulose can provide an effective way for ion transport. The high pore structure and hydrophilic properties of nanocellulose make it easier to transport electrolyte ion [261].
Nanocellulose-Based Paper for Electronic Devices
Nanocellulose-based paper is a green substrate that can be used for electronic and optoelectronic devices. Currently, commercial paper has relatively rough surface and weak mechanical properties which can be quite problematic for electronic device fabrication [269]. Most of the electronic device’s fabrication use non-biodegradable and non-recyclable component such as plastics, glasses and silicones as substrates [270]. Formation of nanocellulose-based paper from NFC via simple filtration method can produce mechanically strong and low coefficient thermal expansion paper as an alternative to commercial paper [6, 269]. According to Li and Lee [270], transparent nanocellulose-based paper for electronic devices has been designed and being applied for electrochromic, touch sensor, solar cells, transistors, organic light-emitting diodes (OLEDs), gravure printing proofer and radio-frequency identification (RFID).
One of the applications of transparent nanocellulose paper is for the production of flexible electronics materials through printing circuit directly on the surface of substrates via coating or thermal deposition techniques. Firstly, the fabrication of flexible electronics was built on special silicon wafer that allows the silicon nanomembranes (Si NMs) to be released and then transferred to NFC substrate with an adhesive layer, and the device was completed by photoresist patterning and dry etching steps as illustrated in Fig. 4 [271].
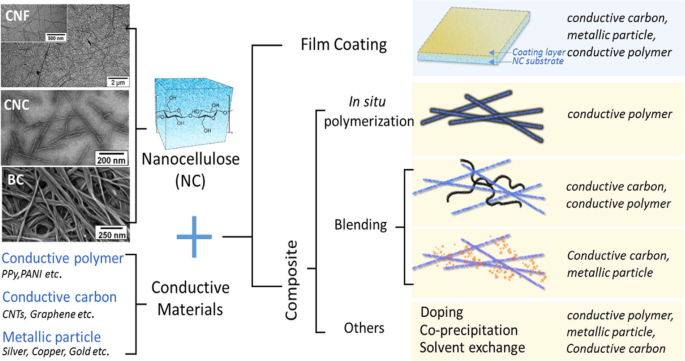
Schematic illustration of the generalised fabrication routes to nanocellulose-based conductive hybrid. Source :Du et al. [237]. Reproduced with permission of Elsevier
Moreover, NFC also is the main substrates for organic light-emitting diodes (OLEDs) (Fig. 5). Okahisa et al. [272] reported that the OLEDs device was fabricated on wood-based nano fibrillated cellulose (NFC) composite.
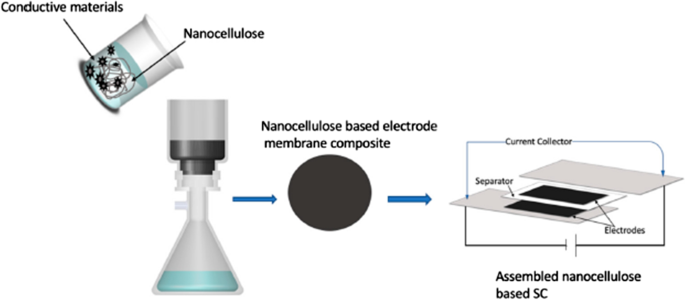
Filtration procedure to fabricate nanocellulose-based composite membrane [supercapacitor (SC)]. Source :Hsu and Zhong [244]
Potential of Nanocellulose-Based Composite for Development of Sensor
Nanocellulose has been widely used to develop novel sensors and improve the sensitivity of sensors. In food industry for example, sensors have become an important tool to protect humans from health hazards and risks caused by the food contaminants. Sensors can help to quickly identify mycotoxins, pathogens, heavy metals, pesticides, metal ions, and so on in food. In addition, sensor technology can overcome the complicated, laborious, and time consuming process using expensive instrument that usually requires well-trained personnel [273, 274]. Sensor also provides rapid and sensitive food safety detection.
In the last decade, plenty of sensors (electrochemical sensors, biosensors and chemical sensors) have been successfully developed as alternative or as complementary detection tools for the rapid and sensitive detection [275,276,277]. However, conventional sensors were developed using plastics, petrochemical-based products and inorganic material which lead to environmental problems. Moreover, issues such as green gas emission, toxicity and sustainability of the materials are becoming increasing. Therefore, the demand for sustainable sensor devices has increased rapidly in recent years.
Nanocelluloses from plants and bacteria have shown promising potentials due to their excellent physical, thermal, mechanical, optical and physical properties, which are important for fabricating high-performance sensor devices. These properties make nanocellulose more preferred as biomaterials since it can enhance the selectivity and sensitivity of sensors for the detection of analytes. On the other hand, the adhesion properties of nanocellulose prevents the leaching problems of immobilised reagents. Thus a remarkable improvement can be obtained for the long-time stability of the sensor. Besides, the hydroxyl –OH groups on nanocellulose can be modified for the incorporation of binding sites for the selective adsorption of different analyte species [278]. The combination of those characteristics makes the nanostructured nanocellulose fibres an ideal building block for conjugation with other functional materials [248, 279, 280] (Table 6).
Development of Biosensors
Kimet al. [286] immobilised enzymes candida rigosa lipase into the different cellulose nanocrystals. The findings indicate candida rigisa lipase absorbed on nanocellulose was relatively higher compared to that of microcellulose. This can be related to the high surface area on nanocellulose and thus increase the ionic interaction between nanocellulose anionic group and candida rigisa lipase. In this study, they found that the half-life of the candida rigisa lipase immobilised in nanocellulose increased 27 times higher compared to in free form. Besides, the stability of enzyme also increased (Fig. 6).
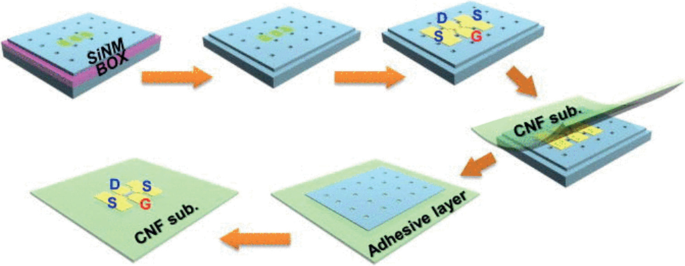
Flexible electronics production process on NFC substrate. Source :Sabo et al. [271]
Another group of researchers, Edwards et al. [287] studied the kinetic profiles of tri- and tetrapeptides substrate of elastase for the fabrication of elastase biosensor whereby known as human neutrophil elastase (HNE) and porcine pancreatic elastase (PPE). To develop HNE and PPE, immobilised tri- and tetrapeptides were used into cotton cellulose nanocrystals. They found that 2 mg of tripeptide conjugated cotton cellulose nanocrystals in 1 h was able to detect 0.03 U mL −1 PPE, while 0.2 mL tetrapeptide conjugated cotton cellulose nanocrystals over 15 min could detect 0.05 U mL −1 HNE activity. Incani et al. [288] fabricated a biosensor by immobilising glucose oxidase (GOx) enzyme in a nanocellulose/polyethyleneimine (PEI)/gold nanparticles (AuNPs) nanocomposites. The AuNPs were adsorbed on the cationic PEI and nanocellulose. The Fourier Transform Infrared (FTIR) spectra confirmed that GOx was successfully immobilised on the polymer composites (Fig. 7).
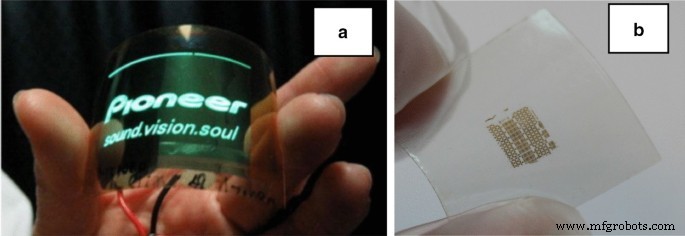
Flexible display (OLEDs) on NFC substrate, a Source :Okahisa et al. [272]. Reproduced with permission of Elsevier. Flexible electronics on NFC substrate, b Source :Sabo et al. [271]
Abd Manan et al. [289] successfully developed biosensor based on nanocrystalline cellulose (NCC)/cadmium sulphide (CdS) quantum dots (QDs) nanocomposites for phenol determination. They modified the NCC with cationic surfactant of cetyltrimethylammonium bromide (CTAB) and further decorated with 3-mercaptopropionic acid (MPA) capped CdS QDs as a scaffold for immobilisation of tyrosinase enzyme (Tyr). The TEM images of NCC and CTAB-NCC (Fig. 8a, b) indicates that agglomerated whiskers-like structure of NCC was not affected by modification, while the MPA QDs are of spherical shape (Fig. 8c). The FESEM images of CTAB-NCC nanostructured film (Fig. 8d) exhibited a homogenous, uniform and dense fibrous structures aggregated, while for CTAB-NCC/QDs nanocomposites film (Fig. 8e), the CdS QDs were appeared as like tiny white dots. EDX analysis shows the presence of respective elemental of carbon (C), oxygen (O), sulphur (S) and cadmium (Cd), indicating the CdS QDs was successfully attached into the CTAB-NCC film. The test finding against phenol shows the biosensor exhibits good linearity towards phenol in the concentration range of 5–40 µM (R2 = 0.9904) with sensitivity and limit of detection of 0.078 µA/µM and 0.082 µM, respectively.
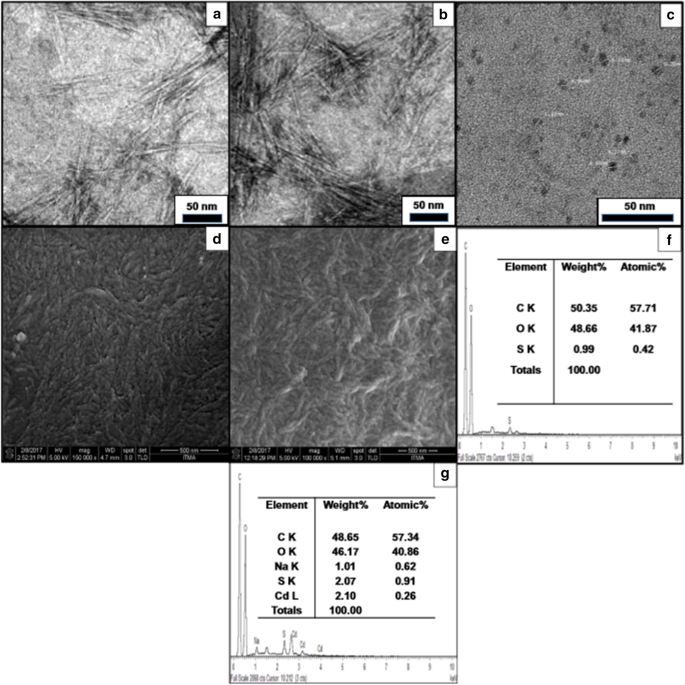
The TEM images for a NCC, b CTAB-NCC, c MPA-QDs, the FESEM micrograph of d CTAB-NCC nanostructures film, e CTAB-NCC/QDs nanocomposites film, f EDX analysis of CTAB-NCC nanostructured film, g CTAB-NCC/QDs nanocomposite film (Source :Abd Manan et al. [289]). Reproduced with permission of Elsevier
In the food industry, sensors are used to monitor the quality or freshness of food. Moradi et al. [290] developed a pH sensing indicator based on bacterial cellulose nanofibres for monitoring freshness of fish. They used carrot anthocyanins as an indicator. The fabricated sensor displayed wide colour differences from red to gray over the 2–11 pH range. The colour changes were distinguishable whereby deep carmine colour indicates fresh fish, charm pink colour indicates fish is best to eat immediately, and spoiled fish is indicated by jelly bean blue and khaki colours.
Development of Chemical Sensors
Nanocellulose hydrogels structure was previously used to immobilise sulphur and nitrogen co-doped graphene quantum dots as a low-cost sensor for detecting the laccase enzyme [291]. Laccase is the multicopper oxidase family of enzyme. It is involved in the monoelectronic oxidation of several aromatic compounds and aliphatic amines. Therefore, it is usually used in decolouration and coloration products [292, 293]. Therefore, monitoring of laccase activity in commercial products is of great interest. From this study, the authors found the sulphur and nitrogen co-doped graphene quantum dots were self-organised via electrostatic interactions.
Faham et al. [294] described the development of a nanocellulose-based colorimetric assay kit for smartphone sensing of iron, Fe(II) and iron-chelating deferoxamine drug (DFO) in biofluids. They embed curcumin in a transparent bacterial cellulose nanopaper, as a colorimetric assay kit. The assay kit was then used for monitoring of iron and deferoxamine as iron-chelating drug in biological fluid such as urine, blood, saliva and serum. The assay kit can be easily coupled with smartphone technology for colorimetric monitoring of Fe(II) and DFO.
Development of Electrochemical Sensors
Burrs et al. [295] demonstrated the electrochemical biosensor-based glucose for the detection of pathogenic bacteria. The conductive paper used for the development of electrochemical sensor was prepared using graphene-nanocellulose composites. Platinum was electrodeposited on graphene-cellulose composites using pulsed sonoelectrodeposition [296]. Then the sensor was fabricated by functionalising the nanoplatinum with glucose oxidase (GOx ) entrapped in a chitosan hydrogel on the conductive paper. They found that the sensor is extremely efficient for use in electrochemical biosensing with a low detection limit for glucose or pathogenic bacteria.
Ortolani et al. [297] developed an electrochemical sensing device using nanocellulose and single-walled carbon nanohorns (SWCNH) for guanine and adenine determination. Both nanocellulose and SWCNH have large surface area, good conductivity, high porosity and chemical stability. The prepared sensor showed highly sensitive and high electrocatalytic activity towards simultaneous determination of guanine and adenine. The sensor also presented a lower limit detection. Another study conducted by Shalauddin et al. [298] used hybrid nanocellulose/functionalised multi-walled carbon nanotubes (f -MWCNTs for development of electrochemical sensing. The sensor for determination of diclofenac sodium in pharmaceutical drugs and biological fluids samples were used. The presence of –OH groups in the nanocellulose was reported to provide more binding sites for different analytes which ensures an axial modulus rearrangement and incorporation of f -MWCNTs.
Toxicity of Nanomaterial
Although nanomaterial offers many good potential to various industrial sectors, its potential hazard to human and environment could not simply be overlooked [299]. The safety or toxicity issue needs to be addressed by conducting a complete study from various angles. Nanotoxicity or the study of nanomaterials’ toxicity can be classified into several areas namely oxidative stress, genotoxicity, and ecotoxicity. In humans, toxicity can occur through pulmonary, oral and dermal routes. Nanomaterials can affect humans mainly during extraction or production, handling, usage and disposal. Inorganic nanoparticles (NPs) such as ZnO and nickel oxide (NiO) are believed to be more toxic compared to organic nano materials such as nanocellulose. Digested organic NPs are less likely to induce toxicity since the by-products of digestion by stomach acid are simple sugars, although in the case of nanocellulose, the lack of enzyme in human gut capable of degrading cellulose results in the cellulose moving through the gut fairly quickly. A 90-day subchronic toxicity study on Sprague Dawley rats conducted by Ong et al. [300] indicated that no systemic toxicity attributable to 4% dietary consumption of fibrillated cellulose was observed. In the same study, a higher concentration of 30% of nanofibrillated cellulose for 72 weeks also indicated no adverse effects. When present in the air, nanoparticles can also have harmful effects to human health [301]. Multi-walled carbon nanotubes (MWCNT) are possibly one of the most studied NPs for toxicity effects. In the study, Poulsen et al. [302] found that both thick and long as well as thin and short MWCNT induced genotoxic potential and long lasting inflammation and can lead to cardiovascular disease. In contrast, a study on inhalation NCC toxicity in albino rats was conducted and showed no undesirable effects were observed at a maximum concentration of 0.26 mg/L for a period of four (4) hours [303]. At cellular level, cytotoxicity on NCC on different cell types has been studied with most studies concluded that NCC to be non-toxic to a variety of mammalian cells at concentrations below 0.25 mg/L, but can exhibit cytotoxic effects at concentrations of 0.5 mg/L and above [304,305,306,307,308,309]. Primary nanogenotoxicity refers to the possibility of nanoparticles or their by-products to damage DNA directly or indirectly. Secondary genotoxicity is caused by immune system interaction to disturbances cause by nanoparticles. A study by Akerlund et al. [310] indicated that inorganic NPs of Ni and NiO-induced cytokines inflammations which led to secondary genotoxicity in human lung epithelial cells. However, they require further investigation to understand the factors causing this secondary genotoxicity and whether such results are caused by other nanoparticles as well.
NanoTiO2 in wood coatings has been reported to have low toxicity to terrestrial organisms, and no bioaccumulation was observed [311]. Another study conducted on cerium oxide (CeO2 ) nanoparticle or ceria showed that no acute toxicity was observed for Daphnia magna and Thamnocephalus platyurus and D. rerio embryos at test concentrations of 1000, 5000, and 200 mg/L, respectively. However, the same study indicated that a significant chronic toxicity on P. subcapitata was observed with 10% effect concentrations (EC10s) between 2.6 and 5.4 mg/L [312]. Pulido-Reyes et al. [313] explained that the redox cycling between Ce 3+ and Ce 4+ is a unique chemistry of nanoceria which depends on the prevailing environmental conditions. They concluded that in most cerium oxide NP studies, the amount of surface Ce 3+ correlates with toxicity and this can be overcome by blocking the Ce 3+ sites with anions such as phosphates (PO4 3− ) which will reduce reactive oxygen species levels and overcoming oxidative stress. During and after disposal, there is a likelihood that NPs can interact with the environment, whether in soil or aquatic environment. The oxidative stress or carbon-based nanoparticle-induced toxicity is primarily of concern as this condition will affect the oxygen balance in the environment. Wanget al. [314] showed that NCCs’ activated oxidative stress in aquatic organisms (Scenedesmus obliquus algae, planktonic crustacean Daphnia magna and freshwater fish larva Danio rerio) at concentrations above 0.1 mg/L. The NCC form, morphology and concentration are believed to be the contributing factors, thus suggesting evaluation of nanocellulose ecotoxicological impacts when used at large scales. One method of reducing the undesired effect to the environment is by providing a mechanism to capture, retain or recycle the NPs during processing. Magnetic NPs (e.g. magnetite) [315] or surface modification can be used for this purpose. Almost all toxicity studies on nanoparticles recommend more research to be conducted on finding suitable analytical techniques and protocols in detecting organic NPs within biological matrices as well as mechanistic and environmental investigations to understand potential NPs risks on the ecosystem [301, 302, 310, 316].
Conclusions
The potential of nanotechnology in wood-based products sector is enormous. Nanotechnology offers the opportunity to change the landscape of wood-based products industry locally and globally. Forest itself via forest plantation can provide sustainable sources towards the creation of new generation of cellulose called nanocellulose that could offer myriads of application in various industrial fields. Besides, nanocellulose is less expensive than carbon-based material (e.g. carbon nanotube, graphene), environmentally friendly and may improve the recyclability and performance of countless products. For instance, nanocellulose can be used to improve the performance of paper and reduce the use of pulp in papermaking, thus resulting in cost saving. Nanocellulose can also be made into nanopaper or thin film for use in paper packaging or other sector such as electronics which offers alternative to non-renewable plastic. Nanocellulose used in wood composite can lead to the development of advanced composites which can be tailored to specific uses. Together with their strength properties and affordability makes them a viable solution to reduce the need for solid wood. Industrial sectors other than wood-based products could also benefit from the use of nanocellulose such as in energy device and sensors. Various nanocellulose-based products for energy storage and energy harvester applications as well as sensor have been developed at laboratory scale. In view of this interesting development, Malaysia has embarked on the production of nanocellulose from various tropical lignocellulosic materials. Unfortunately, the application of nanocellulose at commercial scale in Malaysia is still non-existent. Thus, it requires collective, concert and intensive efforts from various players that include stakeholders, industry and researchers to promote the use of nanocellulose and ensure that nanocellulose could enter the next level of application and commercialisation stage. The use of nanosized material other than nanocellulose has already benefited and also given advantage to wood-based products area which includes pulp and paper, wood composite, wood coating and wood preservative.
Verfügbarkeit von Daten und Materialien
Alle Daten und Materialien stehen uneingeschränkt zur Verfügung.
Abkürzungen
- AgNPs:
-
Silver nanoparticles
- Al2 O3 :
-
Aluminium oxide
- AuNPs:
-
Gold nanparticles
- BTZ:
-
Benzotriazole
- C:
-
Kohlenstoff
- CCA:
-
Chromated copper arsenate
- Cd:
-
Cadmium
- CdS:
-
Cadmium sulphide
- CeO2 :
-
Cerium oxide
- CH2 CHSi(OC2 H5 )3 :
-
Vinyltriethoxysilane
- CMC:
-
Carboxymethyl cellulose
- CNT:
-
Kohlenstoff-Nanoröhrchen
- CO2 :
-
Kohlendioxid
- CTAB:
-
Cetyltrimethylammoniumbromid
- CTAB-NCC:
-
Cetyltrimethylammonium bromide-nanocrystalline cellulose
- CWA:
-
Castor-oil-based waterborne acrylate (CWA)
- DFO:
-
Deferoxamine drug
- FESEM:
-
Feldemissions-Rasterelektronenmikroskopie
- f -MWCNTs:
-
Functionalised multi-walled carbon nanotubes
- FTIR:
-
Fourier transform Infrared
- GOx:
-
Glukoseoxidase
- HALS:
-
Hindered amine light stabilizers
- HNE:
-
Human neutrophil elastase
- ISE:
-
Inorganic solid electrolyte
- LDH:
-
Layered double hydroxide
- LIBs:
-
Lithium-ion battery
- LiTFSI:
-
Bis(trifluoromethanesulphonyl)imide lithium salt
- L-NCC:
-
Lignin-coated nanocrystalline cellulose (L-NCC)
- MC:
-
Moisture content
- Mg–Al LDH:
-
Magnesium–aluminium layered double hydroxide
- MPA:
-
3-Mercaptopropionic acid
- MUF:
-
Melamine-urea formaldehyde
- MWCNT:
-
Multi-walled carbon nanotube
- NCC:
-
Nanocrystalline cellulose
- NFC:
-
Nanofibrillated cellulose
- Ni:
-
Nickel
- NiO:
-
Nickel oxide
- NPs:
-
Nanopartikel
- NW:
-
Nanowollastonite
- O:
-
Oxygen
- OH:
-
Hydroxyl
- OLEDs:
-
Organische Leuchtdioden
- PA:
-
Polyamide
- PCL:
-
Polycaprolactone
- PCP:
-
Pentachlorophenol
- PDMS:
-
Polydimethylsiloxan
- PE:
-
Polyethylen
- PEG:
-
Poly(ethylene glycol)
- PEO:
-
Polyethylenoxid
- PHB:
-
Poly(3-hydroxybutyrate-co-4-hydroxybutyrate)
- PHRR:
-
Peak heat release rate
- PLA:
-
Polylactic acid
- PMC:
-
Perfluoroalkyl methacrylic copolymer (PMC)
- PMMA:
-
Poly(methylmethacrylat)
- PO4 3− :
-
Phosphates
- POTS:
-
Perfluoroalkyltriethoxysilane
- PP:
-
Polypropylen
- PPE:
-
Porcine pancreatic elastase
- PPG:
-
Propylene glycol
- PS:
-
Polystyrol
- PSU:
-
Polysulphone
- PU:
-
Polyurethane
- PV:
-
Photovoltaik
- QDs:
-
Quantenpunkte
- RFID:
-
Radio-frequency identification
- S:
-
Sulphur
- SC:
-
Supercapacitor
- Si NMs:
-
Silicon nanomembranes
- SiO2 :
-
Siliziumoxid
- SPE:
-
Solid polymer electrolyte
- SPI:
-
Soy protein isolate
- SWCNH:
-
Single-walled carbon nanohorns
- TBOT:
-
Tetrabutyltitanate
- TEM:
-
Transmissionselektronenmikroskopie
- TEMPO:
-
2,2,6,6-Tetramethylpiperidin-1-oxyl
- Ti(OC4H9)4:
-
Tetrabutyltitanate
- TiO2 :
-
Titandioxid
- TMI-BNT:
-
Transition metal ion-modified bentonite
- TNFC:
-
TEMPO-oxidised nanofibrillated cellulose
- Tyr:
-
Tyrosinase
- UF:
-
Urea formaldehyde
- UP:
-
Unsaturated polyester
- UV:
-
Ultraviolett
- VRFBs:
-
Vanadium redox flow batteries
- VTES:
-
Vinyltriethoxysilane
- WPU:
-
Waterborne polyurethane coating
- WUV:
-
Waterborne
- Zn-Al LDH:
-
Zinc-aluminium layered double hydroxide
- ZnO:
-
Zinkoxid
- ZnSt2 :
-
Zinc stearate
- ZnSt2 /WUV:
-
Zinc stearate/waterborne UV
Nanomaterialien
- Anwendung von Molybdän in der Glasindustrie
- Anwendung von Niob in der Stahlindustrie
- Anwendung von Titan in Uhren
- Anwendung von Titan in der pharmazeutischen Industrie
- Pigmente in der Papierindustrie
- Industrie 4.0 Anwendung in verschiedenen Branchen
- Automatisierung in der Industrie 4.0
- Lkw-Industrie in den USA – Anwendung bei der Beschaffung von Gebrauchtmaschinen
- Anwendung des 3D-Drucks in der Automobilindustrie
- Die hydraulische Reparatur-, Umbau- und Wiederaufarbeitungsindustrie:Teil Zwei