Fortschritte und Herausforderungen fluoreszierender Nanomaterialien für die Synthese und biomedizinische Anwendungen
Zusammenfassung
Mit der rasanten Entwicklung der Nanotechnologie sind in den letzten zwei Jahrzehnten neue Arten von fluoreszierenden Nanomaterialien (FNMs) entstanden. Die Nanometerskala verleiht FNMs einzigartige optische Eigenschaften, die bei ihren Anwendungen in der Biobildgebung und fluoreszenzabhängigen Detektionen eine entscheidende Rolle spielen. Da jedoch die geringe Selektivität sowie die geringe Photolumineszenz-Effizienz fluoreszierender Nanomaterialien ihre Anwendung in der Bildgebung und Detektion in gewissem Maße behindert, suchen die Wissenschaftler noch nach der Synthese neuer FNMs mit besseren Eigenschaften. In diesem Aufsatz wird eine Vielzahl von fluoreszierenden Nanopartikeln zusammengefasst, darunter Halbleiter-Quantenpunkte, Kohlenstoffpunkte, Kohlenstoff-Nanopartikel, Kohlenstoff-Nanoröhren, Graphen-basierte Nanomaterialien, Edelmetall-Nanopartikel, Siliziumdioxid-Nanopartikel, Phosphore und organische Gerüste. Wir beleuchten die jüngsten Fortschritte der neuesten Entwicklungen bei der Synthese von FNMs und deren Anwendungen im biomedizinischen Bereich in den letzten Jahren. Darüber hinaus wurden die wichtigsten Theorien, Methoden und Grenzen der Synthese und Anwendung von FNMs besprochen und diskutiert. Darüber hinaus werden auch Herausforderungen in der Synthese und biomedizinischen Anwendungen systematisch zusammengefasst. Die zukünftigen Richtungen und Perspektiven von FNMs in klinischen Anwendungen werden ebenfalls vorgestellt.
Einführung
Herkömmliche organische Farbstoffe haben aufgrund ihrer inhärenten Mängel wie Zytotoxizität und schlechter Biokompatibilität einige Schwierigkeiten bei ihrer Anwendung in der Biomedizin [1]. Das Aufkommen fluoreszierender Nanomaterialien zeigt jedoch großes Potenzial als Ersatz für konventionelle organische Farbstoffe. Wissenschaftler haben viel Zeit und Mühe in die Erforschung fluoreszierender Nanomaterialien investiert, und die diesbezüglichen Errungenschaften in Bezug auf Synthese und Anwendungen sind mehr als inspirierend.
Form, Größe und Struktur fluoreszierender Nanomaterialien bestimmen ihre physikalischen und chemischen Eigenschaften, die einen großen Einfluss auf ihre Leistung haben. Daher ist die kontrollierbare Synthese fluoreszierender Nanomaterialien zu einem heißen Forschungsthema geworden. Die optimalen experimentellen Synthesebedingungen tragen zur am besten geeigneten Größe, Morphologie und Stabilität von fluoreszierenden Nanomaterialien bei. In den letzten Jahren wurden viele Anstrengungen unternommen, um die Biokompatibilität von fluoreszierenden Nanomaterialien durch verbesserte Synthesemethoden zu verbessern [2]. Metallionen wurden in der Vergangenheit üblicherweise mit Kohlenstoffpunkten (CDs) oder Quantenpunkten (QDs) dotiert, um die Oberfläche fluoreszierender Nanomaterialien zu funktionalisieren. Die ineffektive Fluoreszenz und die zugrunde liegende Toxizität stellten jedoch eine Bedrohung für ihre Anwendungen in der Biobildgebung und Biomarkierung dar [3]. Angesichts dieser Probleme haben Zuo et al. berichteten über ein hocheffizientes CDs-Genabgabesystem. Mit Fluor dotierte CDs wurden durch einen solvothermalen Prozess synthetisiert und positive Ladungsstellen für die Genübertragung können durch verzweigtes Polyethylenimin (b-PEI) bereitgestellt werden [4]. Es ist zu erwarten, dass neue Methoden der Oberflächenmodifikation in Zukunft ein Hotspot der Forschung sein werden.
Es wurden viele Anstrengungen unternommen, um das Potenzial fluoreszierender Nanomaterialien für biomedizinische Anwendungen zu erkunden, die Bioimaging, Biodetektion und einige Therapiemethoden umfassen, wie in Abb. 1 gezeigt. Eine zuverlässige Fluoreszenz für die Anwendung hängt von ihren physikalischen und chemischen Eigenschaften ab [5]. Daher war die Forschungsarbeit zur Verbesserung ihrer Eigenschaften wie Toxizität, Hydrophilie und Biokompatibilität ein wesentlicher Bestandteil bei der Realisierung der umfassenden Verwendung fluoreszierender Nanomaterialien in biomedizinischen Bereichen. Mit der steigenden Rate einiger Krankheiten wie Krebs besteht eine zunehmende Nachfrage nach neuen Diagnose- und Therapiestrategien mit höherer Genauigkeit und Compliance von den Patienten [6]. Gegenwärtig sind die Dotierung mit Metall- oder Nichtmetallionen und die Oberflächenmodifizierung von fluoreszierenden Nanomaterialien noch die dominierenden Techniken zur Verbesserung ihrer PL-Effizienz und Biokompatibilität [7], und entsprechende Forschungen eröffnen neue Visionen der biomedizinischen Anwendungen fluoreszierender Nanomaterialien.
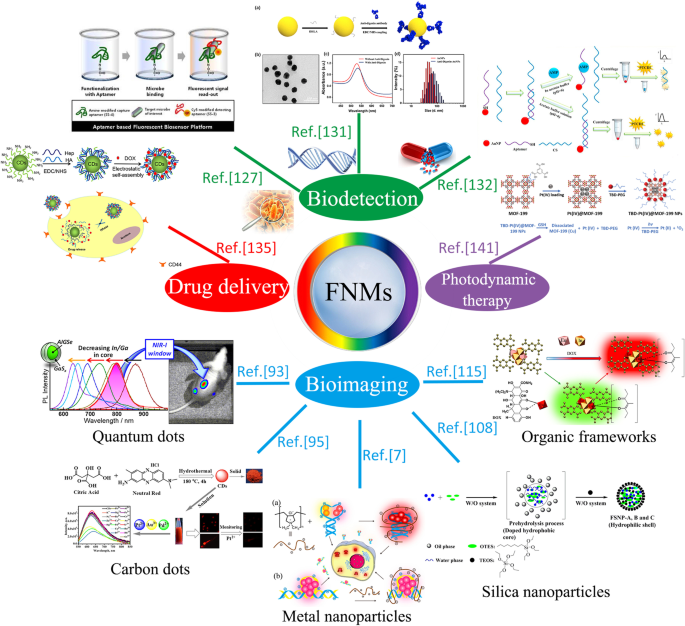
Das Übersichtsdiagramm biomedizinischer Anwendungen fluoreszierender Nanomaterialien
Angesichts des großen Potenzials, das fluoreszierende Nanomaterialien im biomedizinischen Bereich besitzen, legt dieser Aufsatz einen Schwerpunkt auf die neuesten Fortschritte und Verbesserungen. Wissenschaftler haben sich der Funktionalisierung der Oberfläche fluoreszierender Nanomaterialien und deren Leistungen in biomedizinischen Anwendungen gewidmet. Nur mit vernünftig konzipierten Synthesestrategien können fluoreszierende Materialien mit der Qualität hoher PL-Effizienz und guter Biokompatibilität ausgestattet werden, die für ihre Anwendungen im biomedizinischen Bereich unerlässlich sind. Wir hoffen, dass dieser Aufsatz über die Synthese und Anwendungen fluoreszierender Materialien den Lesern helfen kann, den allgemeinen Entwicklungstrend von fluoreszierenden Nanomaterialien derzeit zu verstehen.
Synthese fluoreszierender Nanomaterialien
Quantenpunkte (Halbleiterkristalle)
Quantum Dots (QDs) waren in den letzten Jahrzehnten aufgrund ihrer breiten Absorptions- und symmetrischen Photolumineszenzspektren, ihrer hohen Quantenausbeute, ihrer hohen Beständigkeit gegen Photobleichen, hoher molarer Extinktionskoeffizienten und großer effektiver Stokes-Verschiebungen der Forschungsschwerpunkt [8]. In Bezug auf den Bildungsmechanismus von QDs zeigen Halbleiter dramatische Quantengrößeneffekte, die zu einer Verschiebung des Absorptionsspektrums und des Fluoreszenzspektrums führen, wenn Ladungsträger (Elektronen und Löcher) durch Potentialbarrieren auf bestimmte Bereiche beschränkt werden. Die kleinen Bereiche sind kleiner als die de Broglie-Wellenlänge der Ladungsträger, oder der Nanokristalldurchmesser beträgt weniger als das Doppelte des Bohrschen Radius von Exzitonen im Volumenmaterial [9]. Wenn die Ladungsträger durch Potentialbarrieren in drei räumlichen Dimensionen begrenzt werden, entstehen QDs, die hauptsächlich aus Atomen der Gruppen II-VI (CdSe, ZnS), III-V (GaAs, InP) oder IV-VI (PbS, PbSe).
Über die Synthese von QDs wurde erstmals 1982 berichtet [10, 11]. Nanokristalle und Mikrokristalle von Halbleitern wurden in Glasmatrizen gezüchtet. Mit der Entwicklung fluoreszierender Materialien wurden QDs mit verschiedenen Methoden hergestellt, wie der direkten Adsorptionsmethode, der Linker-unterstützten Adsorptionsmethode, situ-Methoden und der Kombination bisheriger Herstellungsmethoden. Die Kombination bisheriger Verfahren umfasst die Kombination von vorbereiteten Halbleitern mit QD-Vorläufern und die Kombination von zuvor hergestellten QDs mit Halbleitervorläufern, bei denen die Halbleiter oder QDs separat hergestellt werden [12].
Nach einer Reihe von Untersuchungen zur Synthese von QDs berichteten viele Forscher über die Untersuchung der Fluoreszenzeigenschaften von QDs. Bawendiet al. synthetisierten die QDs mit engen Größenverteilungen durch Einführung von Halbleitervorstufen wie Cadmiumsulfid (CdS), Cadmiumselenid (CdSe) oder Cadmiumtellurid (CdTe), um die größenabhängigen optischen Eigenschaften von QDs zu untersuchen [13]. Seitdem ist CdSe die häufigste chemische Zusammensetzung von QDs, und eine Vielzahl von Oberflächenmodifikationen [14,15,16] oder schützende anorganische Hüllen [13, 17] wurden verwendet, um kolloidale Stabilität zu verleihen.
Karbonpunkte
Carbon Dots (CDs) sind aufkommende Nanomaterialien der Nanokohlenstofffamilie mit Größen unter 10 nm, die erstmals 2004 bei der Reinigung einwandiger Kohlenstoffnanoröhren (SWCNTs) durch Elektrophorese gewonnen wurden [18]. Bemerkenswert ist, dass CDs Halbleiter-Quantenpunkte aufgrund ihrer hohen Löslichkeit in Wasser, geringer Zytotoxizität, hoher Photostabilität, anregungsabhängiger Mehrfarbenemission, bevorzugter Flexibilität bei der Oberflächenmodifikation, ausgezeichneter Zellpermeabilität und besserer Biokompatibilität allmählich ersetzen [19, 20]. Im Allgemeinen bestehen die CDs hauptsächlich aus Kohlenstoffquantenpunkten (CQDs) und Graphenquantenpunkten (GQDs). Eine Vielzahl von Synthesemethoden für CDs mit einstellbarer Größe kann grob in zwei Hauptgruppen eingeteilt werden:chemische Methoden und physikalische Methoden [21].
Chemische Synthesemethoden
Chemische Syntheseverfahren werden am häufigsten bei der Herstellung von Kohlenstoffpunkten verwendet, da die resultierenden CDs ausgezeichnete Eigenschaften besitzen, wie beispielsweise eine überlegene Wasserlöslichkeit, chemische Inertheit, geringe Toxizität, einfache Funktionalisierung und Beständigkeit gegenüber Photobleichen. Im Allgemeinen umfassen chemische Synthesemethoden elektrochemische Synthese [22, 23], saure Oxidation [24, 25], hydrothermale Karbonisierung [26], Mikrowellen-unterstützte/Ultraschallbehandlung [27, 28, 29], lösungschemische Methoden [30], unterstützte Synthese [31] usw.
Unter den zahlreichen Synthesemethoden wurde in den letzten Jahrzehnten immer wieder über die elektrochemische Synthese berichtet. Die Gruppe von Zhao berichtete über eine neuartige Methode zur Herstellung von CDs mit geringer Zytotoxizität durch Elektrooxidationssynthese, wobei die CDs durch Oxidation einer Graphitsäulenelektrode gegen eine gesättigte Kalomelelektrode mit einer Pt-Draht-Gegenelektrode in NaH2 . hergestellt wurden PO4 wässrige Lösung [22]. Der Überstand wurde dann durch Zentrifugalfiltervorrichtungen ultrafiltriert, um die CDs mit blauer bzw. gelber Fluoreszenz zu erhalten. Ein weiterer direkter elektrochemischer Ansatz wurde kürzlich von Qu et al. für GQDs mit einer einheitlichen Größe von 3–5 nm durch elektrochemische Oxidation einer Graphenelektrode in Phosphatpufferlösung beschrieben [23]. Die photolumineszente (PL) Farbe dieser Partikel war grün.
Maoet al. schlossen 2007 die Verbrennungs-Oxidations-Synthese von CDs durch Mischen von Kerzenruß mit einem Oxidationsmittel ab, gefolgt von Rückflusserhitzen, Zentrifugieren und Dialyse, um die CDs zu reinigen. Die Photolumineszenzspektren der präparierten CDs haben einen breiten Farbbereich, wobei die Wellenlängen der Emissionspeaks von 415 (violett) bis 615 nm (orange-rot) reichen. Dann wurden die erhaltenen CDs weiter einer Polyacrylamid-Gelelektrophorese unterzogen, um die CDs mit unterschiedlicher optischer Charakterisierung zu trennen. Saure Oxidation wurde auch in großem Umfang für die Herstellung stabiler Nanomaterialien wie Kohlenstoffpunkte verwendet. Nach der Säurebehandlung von Kohlenstoffnanoröhren/Graphit und Rückfluss stellten die resultierenden CDs von 3-4 nm eine hellbraune transparente Flüssigkeit dar, die unter ultraviolettem Licht hellgelbe Fluoreszenz emittiert und in Kochsalzlösung ziemlich stabil war. Dadurch besitzen CDs mit langwelliger (gelb/orange/rot) Fluoreszenz eine bessere Penetration. Die CD-Lösung ist bei Raumtemperatur lange haltbar und es bilden sich keine Präzipitate, die den Fluoreszenzverlust verursachen [25].
Die Mikrowellen-/Ultraschall-Synthese hat sich allmählich und hauptsächlich zu einer synthetischen Hilfstechnologie im Syntheseprozess entwickelt [32]. Fluoreszierende CDs mit einem Durchmesser von 3–5 nm wurden von Xiaos Gruppe über einen wirtschaftlichen, schnellen und grünen Mikrowellen-unterstützten Ansatz synthetisiert [33]. Das hervorstechendste Merkmal dieses einstufigen Ansatzes war, dass sowohl die Bildung als auch die Funktionalisierung von CDs gleichzeitig durch die erstmals aus ionischen Flüssigkeiten abgeleitete Mikrowellenpyrolyse abgeschlossen wurden [34]. Der Reaktionsprozess fand in einem Mikrowellenherd mit billigen ionischen Flüssigkeiten als Kohlenstoffquelle statt und die Lösung änderte sich im Laufe der Reaktionszeit von farblos zu dunkelbraun [35]. Tanget al. verwendeten ein Ultraschallverfahren auf der Basis von Glucose oder Aktivkohle als Kohlenstoffquelle, um monodisperse wasserlösliche CDs zu synthetisieren. Sie emittierten helle und farbenfrohe Fluoreszenz [28]. In ähnlicher Weise haben Vanesa Romero et al. erhielten hochfluoreszierende Stickstoff (N) und Schwefel (S) codotierte Kohlenstoffpunkte (CDs) nach photochemischer Oxidation der Kohlenhydrate in Gemüse. Die Co-Dotierung von N und S erhöht die Anzahl der aktiven Zentren auf der Oberfläche der CDs und verbessert so ihre Lumineszenzleistung [36]. Stickstoffdotierte Kohlenstoffquantenpunkte (NCQDs), eine Fluoreszenzsonde, wurden erfolgreich zur Bestimmung von Doxycyclin eingesetzt [37]. Pathaket al. stellten auch mit Stickstoff und Schwefel kodotierte Kohlenstoffpunkte (NSCDs) her, die aus Thioharnstoff und Tris-Acetat-Ethylendiamin-Puffer durch Mikrowellen-Hydrothermalmethode synthetisiert wurden. Die NSCDs wurden verwendet, um verschiedene pathogene Bakterien und menschliche bukkale Epithelzellen aufgrund von Mehrfarben-Fluorometrie abzubilden [38].
In Anbetracht der Tatsache, dass die meisten der oben genannten Synthesemethoden starke Säuren, mehrere komplizierte experimentelle Schritte und weitere Modifikationen mit anderen Verbindungen erforderten, um die Wasserlöslichkeit der CDs zu verbessern und ihre Photolumineszenz-Eigenschaften zu verbessern, nutzten einige Forschungsteams die hydrothermale Carbonisierung von Kohlenhydrat-Photolumineszenz, wie z Chitosan, Glucose, Citronensäure etc., um die aufwendigen und zeitaufwendigen Reinigungs- und Funktionalisierungsprozesse zu vermeiden [39]. Yanget al. beschrieben eine einstufige Synthesemethode für hoch aminofunktionalisierte fluoreszierende CDs mit einer Quantenausbeute (QY) von 7,8% durch hydrothermale Carbonisierung von Chitosan bei milder Temperatur. Dieses Verfahren benötigt weder ein stark saures Lösungsmittel noch ein Oberflächenpassivierungsreagenz. Außerdem verbesserten die funktionellen Gruppen auf der Oberfläche der CDs ihre Wasserlöslichkeit und reduzierten ihre potenzielle Biotoxizität [26]. Die mehrfach dotierten Kohlenstoffpunkte (MCDs) mit heller und farblich abstimmbarer Emission wurden durch Eintopfverfahren ohne weitere Oberflächenpassivierung synthetisiert. Die synthetisierten MCDs wurden mit reichlich biogenen Elementen (O, N, P) dotiert und zeigen daher eine starke Fluoreszenzemission und eine von der Anregungswellenlänge abhängige Charakteristik, gute Wasserlöslichkeit, hohe optische Stabilität sowie Ionenstabilität. Die MCDs können nicht nur selektiv und sensitiv Fe 3+ . nachweisen unter blauem Detektionslicht bei 15,9 nm, aber auch intrazelluläres Fe 3+ . messen durch Mehrfarben-Fluoreszenzbildgebung [40].
Für lösungschemische Methoden wurde die oxidative Kondensation von Arylgruppen in den letzten Jahrzehnten erfolgreich zur Herstellung von GQDs angewendet. Stabile kolloidale GQDs mit gewünschten Größen und Strukturen wurden von Lis Gruppe mit einer Solubilisierungsstrategie hergestellt. Dieses Verfahren erreichte eine Größenabstimmbarkeit und eine enge Größenverteilung von CDs ohne einen unpraktischen Größentrennungsprozess [30]. Das unterstützte Syntheseverfahren wurde von mehreren Forschungsteams genutzt, um die Synthese monodisperser Nanomaterialien wie nanoskaliger CDs zu vervollständigen. Zhus Gruppe verwendete mesoporöse Kieselsäure(MS)-Kugeln als Nanoreaktoren und Zitronensäure als Kohlenstoffvorstufe und hydrophile CDs mit einer Größe von 1,5–2,5 nm wurden durch eine Imprägnierungsmethode hergestellt. Die CDs mit einer hohen Photolumineszenz-Effizienz von 23 % waren in der Lage, eine starke blaue Lumineszenz zu emittieren und zeigten ausgezeichnete Lumineszenz-Konversionseigenschaften [31]. Hellgelb emittierende Kohlenstoffpunkte (Y-CDs) wurden von Yan et al. durch Solvothermalverfahren unter Verwendung von wasserfreier Zitronensäure als Kohlenstoffquelle und 2, 3-Phenazindiamin als Stickstoffquelle. Die Y-CDs mit zahlreichen Carboxylgruppen zeigten eine respektable Fluoreszenzquantenausbeute (24%), eine Stokes-Verschiebung von 188 nm, eine hohe Empfindlichkeit und ausgezeichnete Stabilität [41]. Die Synthesemethoden und Eigenschaften von CDs sind in Tabelle 1 dargestellt.
Physisch-synthetische Methoden
Im Allgemeinen umfassen physikalische Syntheseverfahren hauptsächlich Lichtbogenentladung, Laserablation/Passivierung und Plasmabehandlung. Xu und Mitarbeiter oxidierten den Lichtbogenentladungsruß mit HNO3 und trennte dann die Suspension durch Gelelektrophorese in SWCNTs. Schließlich isolierten sie das sich schnell bewegende Band von hochfluoreszierenden Kohlenstoff-Punkt-Nanopartikeln [18]. CDs mit Nanokohlenstoffmaterialien als Vorstufe und einem umweltfreundlichen Lösungsmittel als flüssigem Medium wurden von Li et al. über einen milden Laserablationsansatz [44]. Darüber hinaus zeigten Gokus und Mitarbeiter, dass die Verwendung von Sauerstoffplasma die starke Fluoreszenz in einschichtigem Graphen induzieren kann [45].
Kohlenstoff-Nanopartikel
Fluoreszierende Kohlenstoff-Nanopartikel mit ihrer reduzierten Zytotoxizität, Resistenz gegenüber Photobleichen und erhöhter Biokompatibilität ziehen zunehmende Aufmerksamkeit für Bioimaging und andere biomedizinische Anwendungen auf sich. Verglichen mit den typischen Größen von Kohlenstoffpunkten innerhalb von 1–6 nm betragen die Größen von Kohlenstoffnanopartikeln mehr als 20 nm, was das Trennen, Reinigen und Sammeln erspart [46]. Syntheseverfahren für Kohlenstoff-Nanopartikel ähneln Kohlenstoff-Punkten, einschließlich hydrothermale Karbonisierung, Mikrowellenbehandlung, chemische Ablationsverfahren und Laserablation. Diese Methoden haben ihre eigenen Vorteile, können aber die Größe von Nanopartikeln nicht effektiv steuern. Die elektrochemische Karbonisierung ist ein einstufiges Verfahren, mit dem die Größe und die Lumineszenzeigenschaften von Kohlenstoff-Nanopartikeln gesteuert werden können. Leider stehen für dieses Verfahren nur sehr wenige Substrate zur Verfügung. Gegenwärtig wurde über einige neue faszinierende Methoden berichtet, wie z. B. die Phosphorpentoxid-Verbrennungsmethode [47].
In den letzten Jahren werden mit modifizierten Methoden Kohlenstoff-Nanopartikel synthetisiert, die für biomedizinische Anwendungen geeignet sind. Santuet al. lösten die Synthese hochwertiger rot fluoreszierender Kohlenstoff-Nanopartikel durch kontrollierte Karbonisierung von Resorcin [48]. Dieser Ansatz beinhaltet eine oxidative Phenolkupplung, die mit einer Dehydratisierung verbunden ist, um rot fluoreszierende Kohlenstoff-Nanopartikel zu bilden. Anaraet al. synthetisierten fluoreszierende Kohlenstoffnanopartikel mit einer Quantenausbeute von 6.08% unter Verwendung einer modifizierten Hydrothermalmethode. Im Vergleich zu herkömmlichen Methoden, die eine lange thermische Behandlung von bis zu mehreren Stunden erfordern, verkürzte diese Methode die Reaktionszeit auf weniger als 30 Minuten und ermöglichte die schnelle Synthese fluoreszierender Kohlenstoff-Nanopartikel [46].
Kohlenstoff-Nanoröhren
Eindimensionale (1D) Kohlenstoffnanoröhren haben aufgrund ihrer hervorragenden elektronischen und optischen Eigenschaften im biomedizinischen Bereich enorme Aufmerksamkeit erregt. Kohlenstoffnanoröhren können entsprechend der Anzahl der zylindrischen Graphenschichten in einwandige Kohlenstoffnanoröhren (SWCNTs) und mehrwandige Kohlenstoffnanoröhren (MWCNTs) unterteilt werden. Während SWCNTs aus einer einzigen zu einem Zylinder gerollten Graphenschicht bestehen, bestehen MWCNTs aus mehreren konzentrischen Schichten von Graphenfolie. Der Außendurchmesser von Kohlenstoffnanoröhren liegt unter 100 nm, ihre Länge kann jedoch mehrere Millimeter erreichen, was zu einem sehr hohen Aspektverhältnis und einer großen Oberfläche führt [49]. Darüber hinaus bildet die einzigartige Anordnung der Kohlenstoffatome in Kohlenstoffnanoröhren eine reiche π-Elektronenkonjugation außerhalb der Nanoröhre [50]. Darüber hinaus besitzen Kohlenstoffnanoröhren eine starke Absorption und Fluoreszenz im NIR-Bereich [51]. All diese Eigenschaften tragen zu einer effektiven Interaktion mit Biomolekülen bei, was Kohlenstoffnanoröhren zu einem idealen Kandidaten für biomedizinische Anwendungen macht.
Synthetische Methoden haben großen Einfluss auf Durchmesser, Länge, Struktur, Chiralität und Qualität von Kohlenstoff-Nanoröhrchen, und es sollte inzwischen überlegt werden, ob diese Methode für eine großtechnische Produktion geeignet ist. Zu den gebräuchlichsten Methoden gehören die Lichtbogenentladung [52], die Laserablation [53] und die chemische Gasphasenabscheidung [54]. Darüber hinaus müssen Kohlenstoffnanoröhren funktionalisiert werden, um ihre Löslichkeit zu verbessern und ihre Aggregation in Lösungsmitteln und biologischen Medien zu verhindern. Eine kovalente Funktionalisierung würde Defekte in die Struktur von Kohlenstoffnanoröhren einführen, was zu einer dramatischen Abnahme oder sogar zum vollständigen Verlust ihrer NIR-Fluoreszenz führen würde. Eine nichtkovalente Funktionalisierung mit amphiphilen Molekülen wie Polymeren würde die Struktur und die Fluoreszenzeigenschaften von Kohlenstoffnanoröhren erhalten, aber die QY von Kohlenstoffnanoröhren verringern. Um diese Hindernisse zu überwinden, wurden kürzlich neue Methoden zur Synthese und Funktionalisierung von Kohlenstoffnanoröhren beschrieben. Leeet al. berichteten, dass die Zugabe von Dithiothreitol, einem Reduktionsmittel, zum ersten Mal die Fluoreszenz-QY von SWCNTs verstärken kann, was zu Fluorophoren mit einer Helligkeit äquivalent zu der von QDs führt [55]. Hou et al. untersuchten die Addition von Dithiothreitol an SWCNTs, die mit einer Vielzahl von Tensiden funktionalisiert wurden. Bei DNA- und SDS-umhüllten SWCNTs stieg deren Fluoreszenz-QY signifikant an, während bei anderen Tensiden eine Fluoreszenzlöschung in unterschiedlichem Ausmaß beobachtet wurde [56]. Infolgedessen sind die Zugabe von Dithiothreitol zu DNA- oder SDS-umhüllten SWCNTs praktikable Lösungen, um die Anwendung von Kohlenstoffnanoröhren in der Biomedizin zu erreichen.
Graphenbasierte Nanomaterialien
Als zweidimensionale Kohlenstoff-Nanomaterialien wurden Graphen und seine Derivate für eine Reihe biomedizinischer Anwendungen wie Bioimgaing und Wirkstofffreisetzung umfassend erforscht. Graphen-Nanomaterialien umfassen Graphen-Nanoblätter, Graphenoxid (GO) und reduziertes Graphenoxid (rGO)-Nanoblatt. Sie haben große Oberflächen und einzigartige Oberflächeneigenschaften, die nichtkovalente Wechselwirkungen mit Farbstoffmolekülen, Biomolekülen und wasserunlöslichen Arzneimitteln ermöglichen. Viele Forscher haben über verschiedene Verfahren zur Herstellung von Graphen berichtet, seit es 2004 zum ersten Mal erfolgreich hergestellt wurde. Synthetische Verfahren von Graphen-Nanomaterialien können in zwei Kategorien eingeteilt werden, die Top-Down- und die Bottom-Up-Methode.
Top-down-Methoden beinhalten die Isolierung von gestapelten Graphitschichten, um Graphenschichten zu bilden, einschließlich mechanischer Exfoliation [57], lösungsmittelbasierter Exfoliation [58] und elektrochemischer Exfoliation [59]. Guet al. untersuchten systematisch ultraschallunterstützte Peelings auf Lösungsmittelbasis und fanden heraus, dass Ultraschallwellen eine gute Peelingwirkung haben. Sie können auch die Größen- und Dickenverteilung von Graphenschichten beeinflussen, was eine kontrollierbare Synthese ermöglicht. Bottom-up-Ansätze beinhalten die Reorganisation von Kohlenstoffatomen unter Verwendung alternativer Kohlenstoffquellen. Epitaxiales Wachstum [60] und chemische Gasphasenabscheidung (CVD) [61] sind die am häufigsten verwendeten Bottom-up-Synthesemethoden. GO-Blätter bestehend aus vielen sp 2 durch sauerstoffhaltige Gruppen isolierte Domänen können mit der Hummer-Methode synthetisiert werden. Variationen in den Größen dieser sp 2 Domänen führen dazu, dass der PL von GO-Blättern weit von 500 bis 800 nm reicht [62]. rGO wird aus GO durch chemische Reduktion mit Reduktionsmitteln wie Hydrochinon und Hydrazin gewonnen. Im Vergleich zu GO zeigte die Fluoreszenz von rGO eine blauverschobene Emission im UV-Bereich zusammen mit einer Fluoreszenzlöschung, was auf die Perkolationspfade zwischen den neu gebildeten kristallinen sp 2 . zurückgeführt wird Cluster [63]. Akbariet al. verdeutlichte, dass das Verhältnis von sp 3 /sp 2 Domänen in GO-Faltblättern bestimmt ihre Fluoreszenzspektren. Daher ist GO ein vielversprechendes fluoreszierendes Nanomaterial über einen weiten Wellenlängenbereich mit unterschiedlichen Reduktionsgraden, das in biomedizinischen Anwendungen verwendet werden kann.
Metallische Nanomaterialien
Edelmetallatome weisen im Vergleich zu QDs gleichzeitig eine geringere Zytotoxizität auf. Gold-, Silber- und Kupfer-Nanopartikel haben zunehmende Aufmerksamkeit erfahren und werden in einer Vielzahl von Bereichen eingesetzt. Im biomedizinischen Bereich machen die quantenmechanischen Effekte von Goldnanopartikeln, wie Photolumineszenzemission oder Plasmonenresonanz, Goldnanopartikel (AuNPs) zu einem idealen Kandidaten für einen weiteren In-vivo-Nanosensor mit geringer Zytotoxizität [64, 65].
AuNPs haben aufgrund ihrer einfachen Synthese und einzigartigen Eigenschaften großes wissenschaftliches Interesse auf sich gezogen, und es wurden verschiedene Synthesemethoden beschrieben. Als eines der wichtigsten Verfahren werden chemische Verfahren im Allgemeinen durch Behandeln einer wässrigen Lösung von Chloraurat mit Reduktionsmitteln in Gegenwart eines Stabilisierungsmittels durchgeführt. Am weitesten verbreitet ist Zitronensäure, die sowohl als Stabilisator als auch als Reduktionsmittel wirken kann [66]. Mit Zitronensäure stabilisierte AuNPs können jedoch während der Funktionalisierungsentwicklung mit Thiolatliganden eine irreversible Akkumulation durchlaufen. Dieses Problem kann überwunden werden, indem man die Reaktion in Gegenwart von wasserlöslichen Polymeren, Tensiden oder Verkappungsmitteln ablaufen lässt, die zu einer höheren Stabilität beitragen und die Aggregation von Nanopartikeln verhindern. Größe und Form von AuNPs können durch Änderung des Gold-Citrat-Anteils, Oberflächenmodifizierungsmittel oder Reaktionsbedingungen gesteuert werden. Mit der Eintopf-Ultraschallemulgierungsmethode haben Zhang und seine Mitarbeiter Bis(4-(N-(2-naphthyl)phenylamino)phenyl)-fumaronitril und AuNPs gemeinsam in Mizellen geladen, um die Nanosonde zu erhalten [67]. Vor allem die erhaltene Nanosonde mit großem Potenzial für die Anwendung in der tumorgerichteten Bildgebung und Diagnose in vivo verarbeitete trotz der Existenz von Goldnanopartikeln eine hervorragende Fluoreszenzbildgebungskapazität. Obwohl AuNPs unter bestimmten experimentellen Bedingungen nicht toxisch sind, müssen die Toxizität und die Nebenwirkungen gründlich untersucht werden [68].
Fluoreszierende Ag-Nanocluster haben aufgrund ihrer einzigartigen physikalischen und chemischen Eigenschaften viel Aufmerksamkeit erhalten. Der Syntheseprozess solcher Nanocluster wird durch das stabilisierende Gerüst in DNA-Oligonukleotide, Peptide, Proteine, Dendrimere und Polymere eingeteilt. Außerdem hat die umfangreiche Literatur einige grüne Synthesen gezeigt, wie die Anwendung wässriger Stängelextrakte von D. trifoliata und S. alba um die Vorbereitungsbedingungen zu optimieren [69].
Cu-Nanocluster (Cu-NCs) werden relativ häufig als Edelmetallmaterialien verwendet, ihre Synthese ist jedoch aufgrund ihrer Anfälligkeit gegenüber Oxidation noch selten. Kürzlich haben Kawasaki et al. stellten erfolgreich stabile Cu-NCs mit einer mikrowellenunterstützten Polyolmethode her [70]. DNA könnte als Matrize für die Synthese von fluoreszierenden Cu-NCs verwendet werden. Mohiret al. schlugen eine Methode vor, die auf doppelsträngiger DNA in Lösung basiert, um Cu-NCs mit hoher Selektivität zu erhalten [71]. Unter Verwendung der Fluoreszenzeigenschaften von Cu-NCs wurde es erfolgreich als effektiver Indikator für ein Fluoreszenz-Einschaltsignal für die selektive Bestimmung von RDX genutzt [72].
Silica-Nanopartikel
Unter Berücksichtigung der Eigenschaften Transparenz, mechanische Stabilität, Robustheit und Stabilisierung der eingebetteten Fluorophore werden Silica-Nanopartikel umfassend in biologischen Bereichen eingesetzt. Zum Beispiel Siliziumdioxid-Kern/Schale-NPs für den intrazellulären Nachweis von Zn 2+ und H2 PO4 − in lebenden Zellen wurden als „off/on“ fluoreszierende Nanosensoren synthetisiert. In den letzten Jahren wurden mit organischen Farbstoffen dotierte Silica-Nanopartikel synthetisiert und in vielen Anwendungen wie der Biodetektion weit verbreitet eingesetzt [73]. Die am weitesten verbreiteten Synthesemethoden für Silica-Nanopartikel sind die Stöber-Methode und die reverse Mikroemulsionsmethode. Die erstmals in den 1960er Jahren beschriebene Stöber-Methode [74] beinhaltet die Hydrolyse von Alkylsilikaten und die anschließende Kondensation von Kieselsäure in alkoholischen Lösungen unter Katalyse durch Zugabe von Ammoniak. Die zweite Methode zur Bildung von Siliciumdioxid-NP, die Umkehrmikroemulsionsmethode, beinhaltet die Reaktion von Alkylsilikaten, typischerweise TEOS, in den Wassertröpfchen einer Wasser-in-Öl-Mikroemulsion [75]. Er et al. stellten drei Arten farbstoffdotierter Siliciumdioxid-Nanopartikel mit der Stöber-Methode und der Umkehrmikroemulsionsmethode her, indem Farbstoff in den Kern des Partikels eingebettet wurde. Einige Moleküle reagieren funktionell auf Zn 2+ werden auf Partikeloberflächen abgeschieden [76]. Die fluoreszierenden Siliziumdioxid-Nanopartikel wurden für fluoreszierende Bilder intrazelluläres Zn 2+ . verwendet (H2 PO4 − ) in HeLa-Zellen. Wenn Zn 2+ wurde zum proportionalen Zn 2+ . addiert Nanosensor zeigten die Nanopartikel die Fähigkeit, die Konzentration von H2 . ratiometrisch zu erfassen PO4 − .
Im Allgemeinen können Silica-Nanokomposite mit guter Monodispersität und Biokompatibilität leicht mit funktionellen Gruppen weiter modifiziert werden [77,78,79]. Leeet al. dotierte magnetische Nanopartikel und fluoreszierende Farbstoffe in Siliziumdioxid-Nanopartikel. Diese Siliziumdioxid-Nanokomposite können nicht nur als multimodale Bildgebungssonden für die Magnetresonanz- (MR) und Fluoreszenzbildgebung verwendet werden, sondern auch als Träger für die Wirkstoffabgabe gegen Krebs [80]. Kurz gesagt, Silica-Partikel könnten in solchen Fällen ein Forschungsgebiet mit stark erweiterter Anwendung sein.
Phosphore
Phosphore werden in der Biomedizin aufgrund ihrer einzigartigen Vorteile bei der Verringerung der Autofluoreszenz und der Lichtstreuungsinterferenz von Geweben weit verbreitet verwendet. Im Allgemeinen bestehen Leuchtstoffe aus Wirtsmaterialien und dotierten Ionen [81]. Unter den Wirtsmaterialien von Leuchtstoffen ist Yttriumoxid (Y2 O3 ) ist aufgrund seiner geringen Photobeständigkeit und seiner Phononenenergie mehr als vielversprechend. Lanthanoide sind in Leuchtstoffen in Anbetracht ihrer reichlich vorhandenen Elektronenniveaus und Energieübertragungskanäle weitgehend dotiert. Für die Herstellung von Leuchtstoffen wurden zahlreiche Methoden beschrieben, darunter hydrothermale [82], Flammsprühpyrolyse [83], Sol-Gel [84] und Co-Präzipitationsverfahren [85].
Als ideales Verfahren entwickelt sich die hydrothermale Synthese, die sich für die Synthese von Leuchtstoffen als effizient und wirtschaftlich erwiesen hat. Yuet al. synthetisiert Y2 O3 :Eu 3+ Phosphore durch hydrothermale Methode in Gegenwart von Natriumcitrat [82]. Das Konzentrat von Natriumcitrat, die Zugabemenge von NaOH und Eu im hydrothermalen Verfahren bestimmten die Eigenschaften der erhaltenen Leuchtstoffe. Die Flammsprühpyrolyse ist eine vielversprechende Methode zur schnellen und konsekutiven Synthese von oxidbasierten Leuchtstoffen. Im Vergleich zu herkömmlichen Verfahren liefert dieses Verfahren Leuchtstoffe mit hoher Kristallinität und homogener Dotierstoffverteilung. Khan et al. erfolgreich produziert Tb 3+ –dotiert Y2 O3 Leuchtstoffe mit einem Durchmesser von ca. 100 nm und einer engen Größenverteilung mittels Flammensprühpyrolyse [83]. Bei ihrem Verfahren wurde Alkalisalz mit anderen Metallnitratvorläufern gemischt, wodurch die Größenverteilung in einem engen Bereich effektiv kontrolliert wurde. The sol–gel synthesis route offers several advantages, such as high homogeneity and purity, reduced synthesis time, uniform particle morphology, and narrow particle size distribution [86]. Leonardo et al. obtained Sm 3+ doped SiO2 -Gd2 O3 phosphors by a sol–gel process [84]. Co-precipitation is a common and simple method for synthesizing crystalline phosphors, which ensures high homogeneity and controlled morphology characteristics. Perhaita et al. reported that the phase composition of the phosphors strongly depends on the pH during the precipitation [85].
Organic Frameworks
Covalent-organic frameworks (COFs) are new porous crystalline materials possessing outstanding stability, adsorption and low toxicity. The design of fluorescent small organic molecules with a combination of fluorescence determination methods can be used to construct more efficient nanoprobes [87]. For selective 2,4,6-trinitrophenol (TNP) determination, a novel Naphthalimide-Benzothiazole conjugate was prepared as colorimetric and fluorescent nanoprobe. The fluorescence emission peaks of receptor were selectively quenched by TNP with a limit of detection as low as 1.613 × 10 –10 M.
Metal organic frameworks (MOFs) are a kind of new generation multifunctional inorganic–organic materials with various holes and functionalized 3D crystalline structures formed by metal ions and linkers. MOFs show potential applications in separation, catalysis and other aspects due to unique attributes such as excellent chemical tenability, specific surface area and confinement of the pores. Some of the MOFs are luminescent and the quantum yield as well as light intensity will be influenced by temperature and excitation wavelength [88]. With the addition of doxycycline, Yu et al. synthesized a new functional metal–organic framework of pyromellitic acid and europium, which exhibited remarkable fluorescence enhancement at 526 nm and 617 nm. Results showed that both fluorescence intensities were positively correlated with the doxycycline concentration. The unique fluorescence response of the system could discriminate doxycycline from other tetracycline antibiotics with high selectivity.
Biomedical Applications of Fluorescent Nanomaterials
Bioimaging
Quantum Dots for Bioimaging
Fluorescent nanomaterials have been widely used in bioimaging. Compared with conventional organic fluorescent molecules, fluorescent nanomaterials are equipped with many superior properties such as high photostability, tunable emission spectra and high quantum yields [89].
As early as 1998, QDs were first successfully applied in biological imaging [90]. Since then, applications of QDs in this field have been springing up gradually. Chen’s group applied it in bioimaging and nuclear targeting with great stability and biocompatibility in living cells [91]. In spite of the extremely high sensitivity and spatial resolution of QDs, poor performances on hydrophilicity and biocompatibility hindered their applications in bioimaging in vivo. To tackle this problem, it has been found that the water-solubility of QDs can be greatly improved by attaching thiol or other hydrophilic groups to the surface of quantum dots [92]. In the same way, with the intention of improving the effectiveness and specificity of in vivo targeted imaging, targeting molecules are attached to the surface of QDs. Furthermore, the wavelength region of the emission light can be controlled by altering the size of QDs.
The combination of QDs and inorganic metal ions can optimize the application of QDs in bioimaging because the QDs’ defect-site PL peaks will be utterly removed by controlling the proportion of doped inorganic metal ions. Kuwabata, S et al. modulated the degree of Ga 3+ doping in Ag–In–Se QDs. Thus, the QDs’ defect-site PL peaks were completely removed and a sharp band-edge emission peak come into appearance [93]. They found a blue shift of the band-edge PL peak ranging from 890 to 630 nm which could be credited to the fact that the energy gap of QDs was enlarged by Ga 3+ doping. After injecting a mouse with QDs, the potential of AIGSe@GaSx core–shell QDs for bioimaging turned out satisfying. The imaging effect of this kind of QDs in mice is demonstrated in Fig. 2. However, sensing of mid-IR wavelengths is challenging due to increased dark currents and noise. HgTe QDs synthesized by colloidal method is a promising candidate for IR bioimaging by virtue of lower dark currents, higher-temperature operation, and higher detectivity [94].
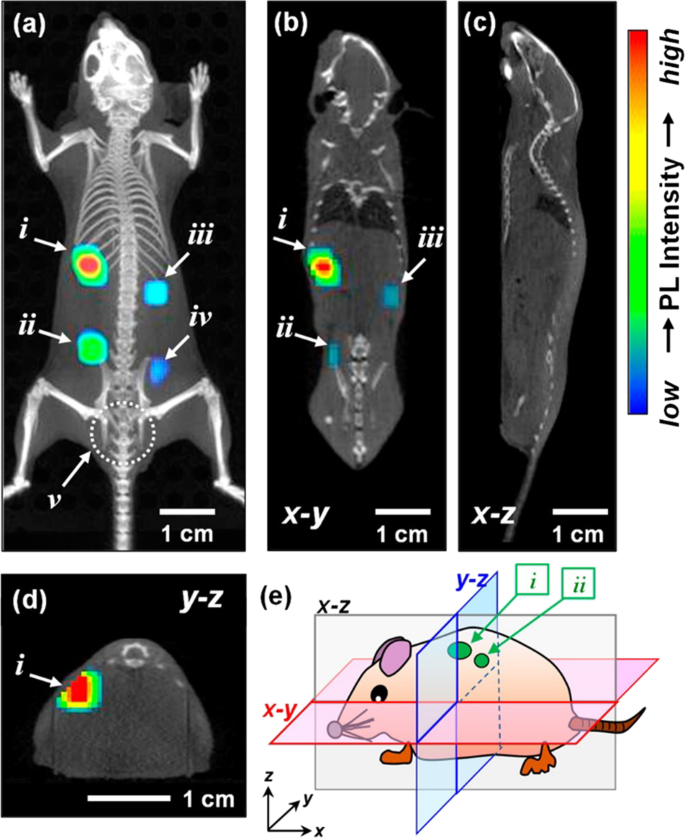
Three-dimensional PL image superimposed on an X-ray CT image of the mouse subcutaneously injected with DSPC-AIGSe@GaSx liposome dispersions (each 50 mm 3 ) in the back [78]
Carbon Dots for Bioimaging
The poor photostability of current fluorescent nanomaterials hinders their long-term bioimaging to a large extent. To overcome this limitation, CDs have been studied for bioimaging and some positive results have been obtained due to the great performance on PL efficiency. Enormous efforts have been put to improve their water solubility and lower their toxicity in organisms. At present, most CDs are facing a barrier in bioimaging, that is, their short-wavelength excitation disables deep penetration in tissue. Aside from this, being exposed under the short-wavelength for a long time could do irreversible damages to living cells and tissues. As shown in Fig. 3, with the purpose of overcoming this deficiency, Gao et al. designed fluorescent CDs with red emission which were successfully used for bioimaging of noble metal ions (Pt 2+ , Au 3+ , Pd 2+ ) in cells and zebrafish [95]. Sun and co-workers first studied the near infrared (NIR) imaging of CDs in vivo using mice as a model. Recently, it was reported that molecules or polymers containing plentiful sulfoxide or carbonyl groups can enhance NIR fluorescence through the surface modification. As shown in Fig. 4, under NIR excitation, sulfoxide or carbonyl groups are bound to the outer layers and the edges of the CDs. Thus, electron transitions are promoted, influencing the optical bandgap [96].
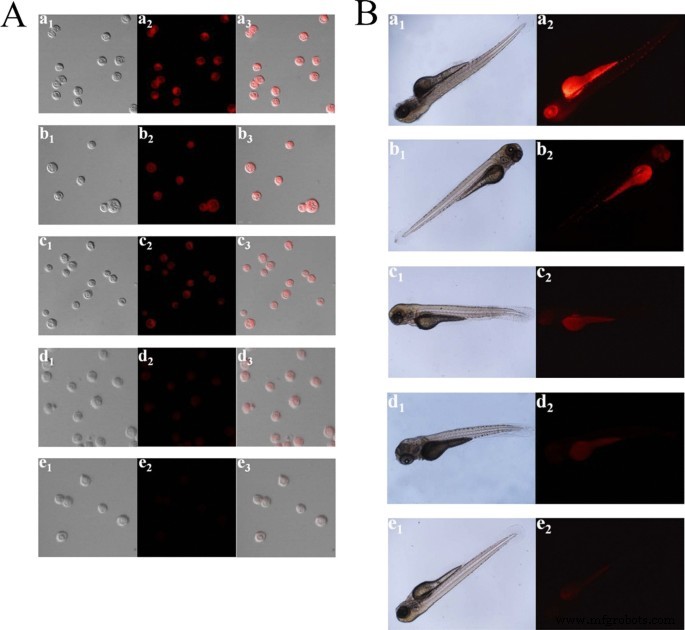
A Confocal imaging of Pt 2+ in PC12 cells. (a1–e1) Bright field images. (a2–e2) Black field images of the CDs in PC12 cells with the different concentrations of Pt 2+ (0, 25, 50, 150, and 300 μM). (a3–e3) Overlay images. B Fluorescence imaging of Pt 2+ in ZF. (a1–e1) Bright field images. (a2–e2) Fluorescence images of the CDs in ZF with the various concentrations of Pt 2+ (0, 30, 60, 100, and 150 μM) [80]
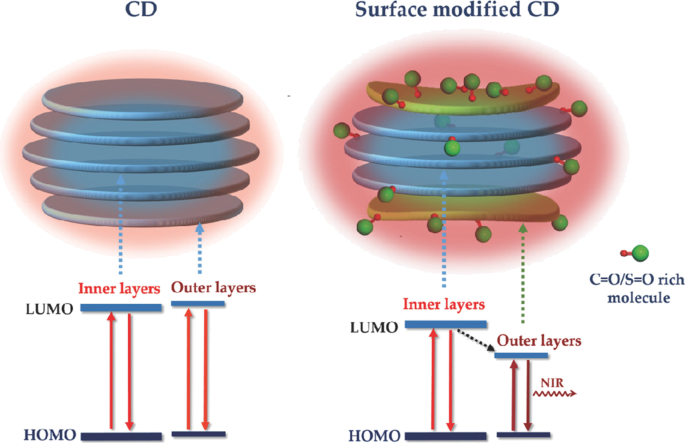
Schematic of structure and energy level alignments of nontreated CDs (left column) and CDs modified with S = O/C = O‐rich molecules (right column). The red (oxygen atom) and green double‐bonded balls represent the C = O/S = O‐rich molecule [81]
Carbon Nanoparticles for Bioimaging
In the field of bioimaging, fluorescent carbon nanoparticles show unique chemical and optical properties over traditional fluorescence probes. Different size, shape and elemental composition make carbon nanoparticles with different features. The biomedical fields are always seeking the most promising fluorescent carbon nanoparticles. Gaurav et al. obtained both larger and smaller size carbon nanoparticles with laser ablation method [97]. Both green and blue fluorescence were observed in the cells incubated with the carbon nanoparticles, suggesting their different sizes. Cell viability results indicated that the prepared carbon nanoparticles were nontoxic and safe for bioimaging applications. Shazid et al. employed carbonization method to obtain fluorescenct carbon nanoparticles derived from biocompatible hyaluronic acid. Both the in vitro and in vivo bioimaging studies showed that the prepared carbon nanoparticles would be reliable and stable for opticle imaging. Moreover, based on the experimenal data, their cytotoxicity was proved to be tolerable for biomedical applications.
Carbon Nanotubes for Bioimaging
Fluorescence of carbon nanotubes in the NIR is attracting high attention for their good light penetration depth in biological tissues. However, their low quantum yield requires for considerable excitation doses, leading to a fair degree of blue-shift and failure of penetrating live tissue. Mandal et al. reported that bright and biocompatible p-nitroaryl functionalized SWCNTs, encapsulated in phospholipid-polyethylene glycol, are suitable for bioimaging applications. The prepared SWCNTs enabled high signal-to-noise ratio imaging in live brain tissues using ultra-low excitation intensities. Their 1160 nm emissions in the NIR guarantee that they will provide optimal fluorescence imaging results [98]. Ceppi et al. applied SWCNT-based fluorescence imaging to debulking surgery in an ovarian cancer mouse model. SWCNTs are coupled to an M13 bacteriophage carrying modified peptide binding to the SPARC protein, which is overexpressed in ovarian cancer, leading to real-time imaging to guide intraoperative tumor debulking. This imaging system enables detection in the NIR window with a pixel-limited resolution of 200 μm, demonstrating real potential in fluorescence imaging guided surguries for patients [99].
Furthermore, fluorescent moieties can be conjugated by a carbon nanotube backbone, which integrate strong fluorescent ability with robust mechanism strength, exhibiting ideal bioimgaing results. Katharina et al. functionalized SWCNTs with an amphiphilic C18 -alkylated polymer conjugated with bright perylene bisimide fluorophores. The polymers wrapping around the SWCNT backbones not only increase their water dispersibility but also promote their biocompatibility by providing a shield. In vitro studies on HeLa cells demonstrated that the biocompatibility of SWCNTs is dramatically improved. In microscopy studies, direct imaging of the SWCNTs' cellular uptake via perylene bisimide and SWCNT emission proved their potential for bioimging [100]. Park et al. combined carbon nanotubes with mussel adhesive proteins which can be specifically targeted at tumors in tissue. They then made carbon nanotubes conjugated with a ZW800 NIR fluorophore to obtain NIR fluorescence imaging [101]. The prepared carbon nanotube probes react with a specific tumor in one hour and can be easily eliminated via urine, demonstrating great value as tumor imaging and detecting agent.
Graphene-Based Nanomaterials for Bioimaging
Large surface area and feasible further functionalization make graphene-based nanomaterials a promising candidate for biomedical applications. However, as a result of their chemical sturctures, graphene nanosheets lack photoluminescence and rGO only display weak fluorescence, which makes it difficult to be utilized in bioimgaing applications. Many researchers attempted to resolve this problem by conjugation of fluorescent dyes and probes onto the large surface of graphene and its derivatives. Sun et al. reported an assembly strategy to prepare fluorescence probe RACD functionalized a single layer GO via π-π interaction and hydrogen bonding. The resluting nanomaterials exhibited that the fluorescent probes reduce the aggregation degree and acquire very well monodispersion, hydrophilicity and photostability, which is attributed to the strong synergy between RACD and GO [102]. Even so, fluorescence quenching remains a critical issue for these materials. In addition, the biocompatibility and toxicity of polymers applied to connect graphene-based materials and fluorescent moieties have not been adequately investigated. These facts appeal for alternative solutions to utilize graphene and its derivatives in bioimaging applications. Georgia et al. developed intrinsically photoluminescent graphene derivatives that show desirable biocompatibility and tunable fluorescence properties [103]. They can be organophilic or hydrophilic with different amine functionalization dodecylamine and hexamethylenediamine, respectively. The intrinsic fluorescent graphene-based nanomaterials possess great potential in a variety fileds of bioimgaing.
Metal Nanomaterials for Bioimaging
In recent years, fluorescent metal nanoparticles have shown great potential in bioimaging for improved disease diagnosis and treatment [104]. Gold is the most commonly used metal for bioimaging. The surface of AuNPs can be easily modified with various biomolecules such as peptides, proteins, antibodies, enzymes, and nucleic acids. These biomolecules can interact with specific cells or organelles in vivo, which makes it possible for AuNPs to be used for targeted optical imaging. Gao et al. reported a real-time in situ imaging of nucleus by AuNPs fabricated with bifunctional peptides constructed with both Au-binding affinity and nucleus-targeting ability. The bifunctional peptides showed strong binding affinity toward AuNPs and ensured good surface coverage of the nanoparticles, which made it stable and efficient for precise bioimaging of the nucleus in cells [105]. The Au-Se bond is considered as a better candidate than the Au–S bond to link the peptides and AuNPs due to the stronger ability against interference of intracellular thiol. Pan et al. prepared the Au-Se-peptide nanoprobes through a direct freezing process. The obtained nanoprobe was successfully applied to identify autophagy and apoptosis in chemotherapeutic drug treated cancer cells [106].
As a novel fluorescent imaging technology, DNA-templated silver nanoclusters (DNA-Ag NCs) have aroused the attention of many scientists due to their unique properties, especially the tunable fluorescence emission range relying on DNA sequences. However, the highly negatively charged DNA backbones have always been a great obstacle for the expansive applications in bioimaging because of poor stability as well as poor cell permeability in physiological environment. It is also noteworthy that the PL property and fluorescent efficiency of DNA-Ag NCs are far from satisfying. As a result, figuring out how to neutralize the negative charge on the surface of DNA strands is of great urgency for researchers. Recently, Lyu and co-workers successfully modified fluorescent DNA-Ag NCs with cationic polyelectrolytes via electrostatic force between the positively charged polyelectrolytes and the negatively charged phosphate groups of the DNA strands, leading to a threefold fluorescence intensity enhancement [7] (Fig. 5). Li et al. reported a facile strategy to make gold nanoclusters with positive charge and silver nanoclusters with negative charge form aggregates by electrostatic interactions. An incredible 40-fold fluorescence intensity enhancement was obtained. Results demonstrated that the physiological stability improved a lot and the cell permeability was also enhanced, which promises its practical applications in the future.
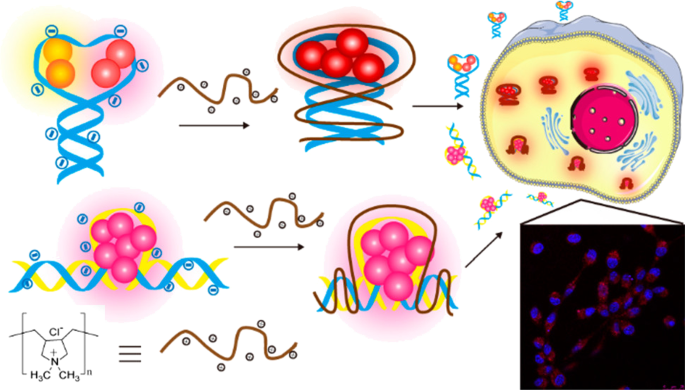
Formation of FL DNA–Ag NC–Cationic Polyelectrolyte Complexes for Cell Imaging [7]
Silica Nanoparticles for Bioimaging
Dye-doped fluorescent silica nanoparticles emerge with great potential for bioimaging as a novel and ideal platform for the monitoring of living cells and the whole body. The outer silica shell matrix protects fluorophores from outside chemical reaction factors as well as provides a hydrophilic shell for the inside insoluble nanoparticles, which renders the enhanced photo-stability and biocompatibility to the organic fluorescent dyes. Benefiting from the robust structure of silica matrices, dye-doped fluorescent silica nanoparticles have been presented with several superior properties including good biocompatibility, hydrophilic features, and high fluorescence intensity [107].
Jiao et al. also constructed a local hydrophobic cage in dye-doped fluorescent silica nanoparticles to improve their optical properties, which solves the problems of aggregation-caused quenching (ACQ) and poor photostability in aqueous media by organic fluorescence dyes benefiting from the robust structure of silica nanoparticles [108]. In addition, compared with free dyes, the fluorescent intensity both in water solution and living cells demonstrated a 12.3-fold enhancement due to the limitation of molecular motion, indicating a significant development for silica nanoparticles in biomedical applications. QDs have been developed for bioimaging both in vivo and vitro owing to their excellent optical qualities. However, a critical obstacle faced in QDs’ application in vivo is their poor biocompatibility. Inspired by the organic dye-conjugated silica-NPs, QDs-embedded silica-NPs have also been invented with the advantage that the excellent optical qualities of QDs can be retained, while the silica-NPs coat improves their biocompatibility to a large extent simultaneously. Darwish et al. reported that many QDs could be assembled around a central silica nanoparticle to form supra-NP assemblies. It was expected to be used for enhanced bioimaging because of their higher sensitivity and superior signal-to-background ratios [109]. There is reason to believe that silica-NPs conjugated with fluorescent nanomaterials with ideal optical properties will still be the dominant research interest in the future.
Phosphors for Bioimaging
For bioimaging, the sizes of phosphors need to be controlled so that they are small enough to be integrated with living cells. Furthermore, the aggregation of particles should be avoided for biocompatibility. Hence, the control of both particle sizes and dispersity in an aqueous solution is essential for the bioimaging application of the phosphors. Atabaev et al. prepared Eu, Gd-codoped Y2 O3 phosphors which had a spherical morphology within the range 61–69 nm. Enhanced PL emission and low toxicity made these phosphors suitable for bioimgaing applications [110].
Upconversion nanomaterials are able to convert lower-energy near-infrared photons to higher-energy ones as emission. This anti-Stokes photoluminescence process will lead to low background noise, large tissue penetration depth, and low photo-damage in bioimaging applications [111]. Lanthanide-based phosphors are able to show upconversion emission owing to their photodurability and low phonon energy. Nallusamy et al. reported a NIR–NIR bioimaging system based on Er 3+ :Y2 O3 phosphors by using NIR emission at 1550 nm under 980 nm excitation, which can allow a deeper penetration depth into biological tissues than ultraviolet or visible light excitation [112]. In addition, the surface of Er 3+ :Y2 O3 was electrostatically PEGylated to improve the chemical durability and dispersion stability under physiological conditions. Thakur et al. synthesized Ho 3+ /Yb 3+ co-doped GdVO4 phosphors via a modified sol–gel method. The prepared phosphors showed brilliant red upconversion emission under NIR excitation, which may be useful in bioimaging of the biomolecules [113].
Organic Frameworks for Bioimaging
Careful selection of MOF constituents can yield crystals of ultrahigh porosity and high thermal and chemical stability, with some of them being luminescent [114]. Recently, Sava Gallis’s group described a novel multifunctional MOF material platform which showed a wide spectral region from 614 to 1350 nm covering the deep red to NIR region. Both porosity and tunable emission properties made them highly suitable for in vivo bioimaging [115]. What’s more, to overcome the obstacle of MOF’s low selectivity towards malignant tissues, Liu et al. developed a target-induced bioimaging by conjugating DNA aptamers using ZrMOF nanoparticles as quenchers [116]. Based on the quenching of ZrMOF nanoparticles, target-induced bioimaging is achieved upon binding with the target.
Biodetection
Since fluorescent nanomaterials can amplify the fluorescent signals significantly and be compatible with organisms, there is increasingly more research on their application in the rapid detection of biomolecules [117]. It will shorten the analysis time to a large extent if we are able to establish a real-time detection system by fluorescent nanomaterials. It has been discovered that multiple detection can be achieved by using QDs probes simultaneously [118, 119].
Pathogen Detection
Pathogens have been an unignorable threat to human health for centuries and these include many types of microorganisms ranging from bacteria (pathogenic Escherichia coli, Salmonella, and Streptococcus pneumoniae ) and viruses (Coronavirus, Influenza virus and hepatitis virus ). However, conventional methods for pathogen detection still need improvement of detection limits and detection speeds. For their applications in detecting pathogens, Tan and co-workers reported a bioconjugated nanoparticle-based biodetection for in situ pathogen quantification, which cost less than 20 min [120]. Tan’s success promises that quick and convenient pathogen detection is possible and can be achieved with these ingenious nanomaterials in the future. Here, we list diverse pathogens detected by fluorescent nanomaterials as shown in Table 2 [119, 121,122,123,124,125,126,127,128,129].
Nucleic Acid Detection
Apart from pathogen detection, fluorescent nanomaterials have also aroused more and more interest of scientists in the detection of DNA. Owing to the merits that a number of biomolecules can be attached to the surface of fluorescent nanomaterials, the signal intensity of fluorescent nanomaterials in DNA detection can be enhanced significantly. Tan and co-workers developed an DNA detection method to detect gene products using bioconjugated dye-doped fluorescent silica nanoparticle with high sensitivity and photostability [130]. Although the analysis of nucleic acid has been successfully achieved by real-time nanomaterial fluorescence systems, there are still many shortcomings such as complex procedures or expensive instruments. To address these disadvantages, Wang’s group introduced a highly sensitive and visualized detection of nucleic acid by the combination of strand exchange amplification (SEA) and lateral flow assay strip (LFA) [131]. The system, which is possible to be widely used in areas requiring limited resource, is mainly characterized by integrating SEA with LFA (Fig. 6). There is no denying that the extremely high fluorescent signal for bioanalysis plays an irreplaceable role in these applications.
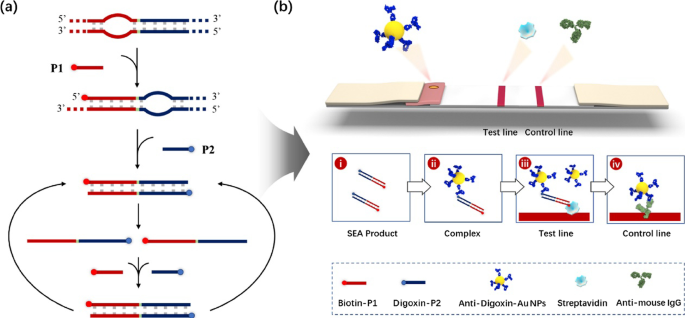
Schematic illustration of the SEA-LFA strip for the detection of nucleic acids [110]
Drug Detection
In the field of drug analysis, a facile and low-cost analytical method is always in demand for high-speed detection of specific pharmaceutical compounds. Real-time detection of drugs can be achieved with selective and sensitive fluorescent nanomaterials owing to their outstanding optical properties. In the past decade, the modification of nanomaterials has lowered their detection limit and improved their detection accuracy significantly. Recently, it is reported that ampicillin can be detected in serum sample based on aptamer, its complementary strand (CS) and gold nanoparticles (AuNPs) [132]. The limit of detection (LOD) of this method can be as low as 29.2 pM. However, there are still many limitations in the detection of drugs or target molecules in vivo. Due to low selectivity, conventional fluorescent nanomaterials inevitably generate false positive results and adverse effects in vivo. In addition, current tracking systems can hardly realize real-time tracking because of insufficient labels and excitation sources. Considering the above limitations, a new method using up/down conversion (UC/DC) PL nanomaterials has attracted increasing attention. Seo et al. reported a single-photon-driven UC/DC system which demonstrated outstanding performance in the detection of heavy metal ion (i.e. Hg 2+ ) in mussels [133]. LOD of the nanohybrids was ca. 1 nM. This system is appealing to researchers in the field of fluorescent nanomaterials for biomedical applications.
Drug Delivery
Until now, the technology of treating cancers with high efficiency and targeting function is not perfect enough. Under most circumstances, the anticancer drugs are distributed and released extensively in the body, which endangers the healthy cells and tissues irreversibly. Currently, a large variety of carriers for drug delivery have been designed. However, we can hardly supervise the distribution and result of the whole delivery process. Benefiting from the recent development of the surface modification technique, fluorescent materials capped with polymers like polyethylene glycol (PEG) can bond with drugs strongly and firmly. Then, the loaded drugs will be released in response to certain conditions such as pH, osmotic gradient and the surrounding environment. However, it should be confirmed whether the drugs are transported to the specific site or not. It’s also necessary for us to consider more details such as how much of the drugs is released in different positions. Aside from being drug carriers, fluorescent nanomaterials can also demonstrate the consequences of intracellular uptake due to their fluorescence property. QDs have been applied to monitor some important properties, such as delivery efficiency, release rate and distribution of drug molecules in vivo, which are beneficial for scientists in order to understand the specific targeting pathways of drug delivery within living cells. Duan and co-workers reported a facile pH-responsive fluorescent CDs drug delivery system [134]. Loaded with dox which is effective for gastric cancer, intracellular drug delivery and tracking could be simultaneously realized in patients (Fig. 7). The report highlighted the ability of fluorescent CDs to label and track the drug delivery process for at least 48 h, which showed a great potential in bioimaging, biolabeling and traceable drug delivery. Duan et al. designed a pH and receptor dual-responsive drug delivery system [135]. Hyaluronic acid was covalently attached to the surface of CDs, and doxorubicin was loaded by electrostatic self-assembly. In the tumor microenvironment (pH 5.6), the drug is released rapidly from the drug delivery system, while in the normal physiological environment (pH 7.4), the drug is hardly released. Endocytosis occurs when the drug delivery system reaches CD44 which is a receptor rich in tumor cells and can bind specifically to the hyaluronic acid. In addition, carbon nanotubes can be used for drug delivery by virtue of their high loading efficiency. Strong π-π interactions play a critical role in binding therapeutic agents with carbon nanotubes, which can be broke through changing external conditions, resulting in the release of drugs in specific position. Pennetta et al. functionalized single and multi-walled carbon nanotubes with a pyrrole derived compound to form a doxorubicin stacked drug delivery system. Biological studies showed that the synthesized nano-conveyors can effectively deliver the drug into cell lines and improve the therapeutic effects of doxorubicin [136].
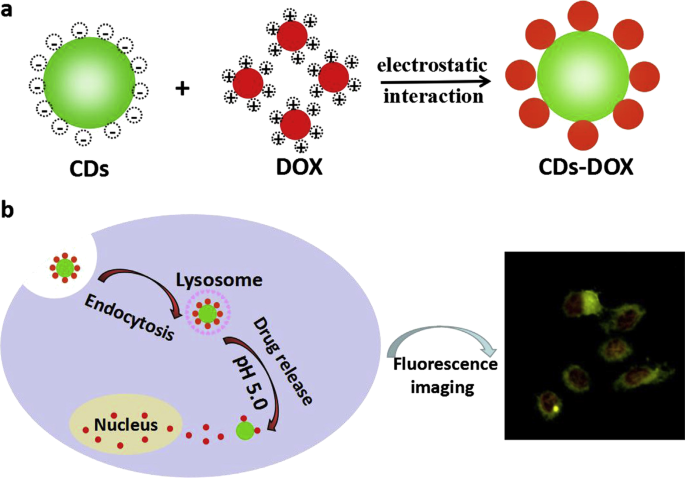
Schematic illustration of the preparation (a ) and cellular uptake (b ) of the CDs-DOX drug delivery system [113]
Photodynamic Therapy
Photodynamic therapy (PDT) is a novel therapy method for tumors which utilizes the interaction between light and photosensitizer. In PDT, reactive oxygen species (ROS) is produced from oxygen by photosensitizers in the condition of specific wavelengths of light (mostly in the area of near infrared light). The specific mechanism is presented in Fig. 8. ROS includes singlet oxygen, superoxide radicals, hydrogen peroxide, and hydroxyl radicals that possess strong cytotoxicity which cause significant destruction of tumor cells. However, there exist many defects such as limited penetration depth [137], hydrophobic properties [138], photobleaching [139], complicated procedure [140], and tumor hypoxia. PDT agents can hardly be dissolved and they will disperse extensively in vivo once they are taken, making it impossible to be targeted and selected. Fluorescent nanomaterial based photodynamic therapy developed fast in recent years [141]. Combined with the unique properties that QDs possess, such as high fluorescent efficiency and great spectral resolution, the effect of PDT can be enhanced. Barberi-Heyob, M and coworkers significantly enhanced the photodynamic efficiency with a concentration of 8 nM because of the light dose-dependent response [142]. In addition, photodynamic therapy can sometimes do harm to the skin and eyes of patients due to its photosensitive side-effect. To alleviate these adverse effects, a novel nanoparticle-based drug carrier for photodynamic therapy is reported which can provide stable aqueous dispersion of hydrophobic photosensitizers. Meanwhile, the key step of photogeneration of singlet oxygen was preserved, which is necessary for photodynamic action [143]. It is obvious that QDs combined photodynamic therapy will replace the conventional PDT someday.
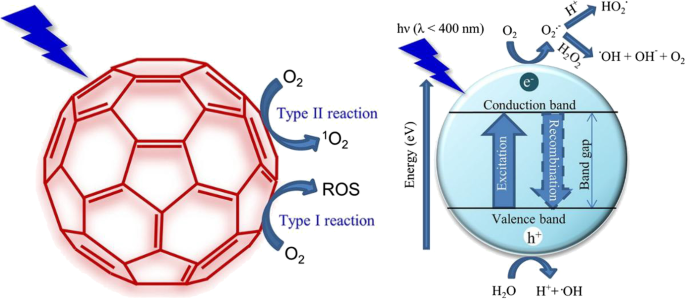
Schematic illustration of producing reactive oxygen species (ROS) for the photodynamic therapy (PDT) [119]
Challenges
Synthesis Challenges
Achieving Uniform Distribution
In the synthesis process, the diameter and size distributions of FNMs can be hardly distributed uniformly due to the agglomeration of small particles. This could be fatal to the optical properties of FNMs in biomedical application. For this reason, the applications of FNMs are still at the laboratory scale. It has been confirmed that the surface properties primarily determine the agglomeration state of the nanoparticles and their size. Therefore, surface modification is promising to achieve uniform distribution of FNMs by altering their surface properties [144]. To date, silanized QDs have been widely used because the polymerized silica coating increases the stability in buffers under physiological conditions [145]. Carbon dots synthesized by hydrothermal reaction using water-soluble base were reported to be difficult to control the size and distribution of grain boundary [146]. Khanam et al. reported a facile and novel synthetic method for the preparation of hydroxyl capped CDs using an organic base and a surfactant (Triton X-100) to modify the surface. A narrow particle size distribution at 7.2 nm was found in Raman and DLS studies, which is smaller than the majority of the particles falling within the range of below 10 nm in diameter [147].
Fluorescence Quantum Yield
Fluorescence quantum yield plays a crucial role for FNMs in their efficiency for on-demand light emission. Tunable and highly fluorescent CDs can be prepared with the surface functionalization approach. Nitrogen-doped FNMs are reported to have improved fluorescence quantum yield. With increasing nitrogen content, fluorescence quantum yield can be increased to as high as 56% at high synthesis temperature [148]. A facile strategy was also developed to tune the photoluminescent properties of CDs using a microwave irradiation, with citric acid and nitrogen-containing branched polyethyleneimine (b-PEI) as precursors. At intermediate levels of b-PEI, the CDs produced a high photoluminescence yield [149]. Lin et al. explored carbon dots with a high-fluorescence quantum yield rate synthesized from L-cysteine and citric acid by the microwave-assisted method. The obtained carbon dots exhibited a high-fluorescence quantum yield (up to 85%), which is due to the combination of amidogens and sulfydryl with carbon dots, and henceforth bringing the improved fluorescence property [150]. The above examples demonstrate that nitrogen or other electron-rich atoms like sulphur can obtain satisfying fluorescence quantum yield.
Aggregation-Caused Quenching
Fluorescent molecules can emit light with high efficiency in dilute solution. However, in concentrated solution or solid state, their fluorescence will be weakened or even disappear. This phenomenon is called Aggregation-Caused Quenching (ACQ) [151]. This problem has been puzzling scientists for almost 150 years, thus hindered the extensive application of fluorescent dyes.
In order to make effective use of fluorescent dyes, scientists have attempted many methods. Most of them focused on reducing the concentration of fluorescent dyes to prevent ACQ effect. Tang et al. discovered the phenomenon of Aggregation-Induced Emission (AIE) [152]. Based on rationally designed molecules, the fluorescence of organic molecules in solid state can be attained. Still, for more than one hundred thousand different fluorescent dyes in the world, the problem of ACQ has not been completely resolved. As long as they aggregate together, ACQ will make them lose their fluorescent properties.
It is almost impossible for high concentration or solid state FNMs to show reliable fluorescence due to fluorescence quenching. Although fluorescence quenching can be used as a sensitive signal to indicate substrate concentration in analytical chemistry, [153] in the most circumstances, however, fluorescence quenching is undesirable for FNMs because it always has considerable influence on bioimaging and biodetection. To overcome this long-standing problem, Benson et al. reported a universal solution with the discovery of a class of materials called small-molecule ionic isolation lattices (SMILES) [154]. SMILES are simple to make by mixing cationic dyes with anion-binding cyanostar macrocycles. We draw inspiration from their findings and believe that similar results can be obtained if we replace cationic dyes with cationic modified FNMs.
Application Challenges
Drawbacks of UV Light FNMs
Although FNMs realized the great-leap-forward from in vitro imaging to in vivo imaging, the emission fluorescence of most of FNMs is distributed in ultraviolet region or short wavelength visible region, which limits the optical imaging in living organisms. Moreover, use of UV light for monitoring living processes in cells and tissues has some potential drawbacks as long‐term irradiation of living cells may cause DNA damage and cell death. Therefore, the development of FNMs in near-infrared region is urgently needed in the future. Although NIR FNMs have deep tissue penetration, NIR detectors and filters are needed as the excitation and emission wavelengths are too close to each other, which restricts their range of application.
Interference in Biological Environment
Almost all biological tissues will produce significant autofluorescence under short wavelength, UV and visible light radiation [155]. Autofluorescence reduces the signal‐to‐background ratio and often interferes seriously with the visual effects. Some substances in the substrate of biological tissues also have great influence on the fluorescence, which reduces the selectivity of FNMs significantly. Until now, although the application of FNMs in mice showed acceptable outcomes, it is still difficult to achieve similar results in larger mammals. Much higher luminous efficiency under low power density excitation is required to avoid the background signal interference. Furthermore, temperature and pH conditions of the biological environments strongly affect the fluorescence of some substances as well. Therefore, satisfying fluorescence of FNMs at 37 °C and physiological pH should be guaranteed. It's worth noting that the pH in tumor is lower than normal tissues. Hence, fluorescence with high selectivity in acid environment will improve the efficiency of FNMs.
Biocompatibility
Biocompatibility refers to materials or systems that are nontoxic, safe and not causing physiological or immunological reactions. QDs with unique quantum confinement effect and electro-optical properties are attractive for biomedical applications. However, toxic effects of traditional semiconductor QDs made of heavy metal ions have serious safety concerns for their undesired environmental or health effects. In the purpose of circumventing this problem, core–shell structure modification of QDs by using biocompatible ligands or polymers is one way to effectively minimize toxic effects of traditional QDs. Furthermore, scientists are searching for heavy metal-free QDs formulations. Non-toxic or less toxic carbon dots and silica nanoparticles have shown their potential as the ideal FNMs for biomedical applications. Impurities brought in the process of syntheses may influence the biocompatibility of fluorescent nanomaterials. In order to reduce the influence of toxic impurities, green synthesis methods have been arousing the interest in biomedical fields. Chowdhury et al. utilized cacao extract which is a natural product as a reducing and stabilizing agent in the synthesis of gold nanoparticles [156]. Oxalic acid, as a constituent of cacao, can reduce Au 3+ in HAuCl4 to metallic gold and stabilize the resultant nanoparticle colloidal solution. In vitro studies suggested that the cacao derived gold nanoparticles are biocompatible and suitable for biomedical applications. For MOFs, appropriate metal ions and ligands must be selected to lower the toxicity. Wang et al. employed Fe, Ti and Zr as constituents of MOFs, which are harmless and even beneficial elements to the body [157]. In vitro studies indicate that the proposed material has good biocompatibility and safety in biomedical application. What’s more, it is necessary to consider whether the difference in composition, surface charge, or modified group will have different biological effects. Taking these factors into account, we can improve the biocompatibility of FNMs with rational design.
Conclusions
Benefiting from the unique properties of fluorescent nanomaterials, some limitations and barriers of conventional materials and methods in biomedical applications can be broken through. In this review, we comprehensively present the synthesis methods and applications of fluorescent nanomaterials. The advanced synthesis methods can offer us the fluorescent nanomaterials with ideal morphology, size ranges and structures. Meanwhile, the more convenient syntheses can lower the manufacturing cost of fluorescent nanomaterials, which is critical to their widespread applications in biomedical fields. Based on the improved synthesis techniques, the performance of fluorescent nanomaterials is bound to leap in their applications. With the development of fluorescent nanomaterials, bioimaging, biodection, drug delivery and photodynamic therapy will be more widely applied in the diagnosis and treatment of diseases. Finally, challenges in synthesis and biomedical applications point out exiting questions and developing direction. We hope that this review can bring some new insights to the development of fluorescent nanomaterials.
Availability of data and materials
All data and materials are available without restrictions.
Abbreviations
- FNMs:
-
Fluorescent nanomaterials
- PL:
-
Photoluminescence
- CDs:
-
Carbon dots
- QDs:
-
Quantum dots
- b-PEI:
-
Branched polyethyleneimine
- SWCNTs:
-
Single-walled carbon nanotubes
- CQDs:
-
Carbon quantum dots
- GQDs:
-
Graphene quantum dots
- NCQDs:
-
Nitrogen-doped carbon quantum dots
- NSCDs:
-
Nitrogen and sulfur doped carbon dots
- QY:
-
Quantum yield
- MCDs:
-
Multi-doped carbon dots
- MWCHTs:
-
Multi-walled carbon nanotubes
- GO:
-
Graphene oxide
- rGO:
-
Reduced graphene oxide
- CVD:
-
Chemical vapor deposition
- AuNPs:
-
Gold nanoparticles
- Ag NCs:
-
Ag nanoclusters
- Cu NCs:
-
Cu nanoclusters
- NPs:
-
Nanoparticles
- TEOS:
-
Tetraethylorthosilicate
- MR:
-
Magnetic resonance
- COFs:
-
Covalent-organic frameworks
- TNP:
-
2,4,6-Trinitrophenol
- MOFs:
-
Metal organic frameworks
- ACQ:
-
Aggregation-caused quenching
- NIR:
-
Near infrared
- SEA:
-
Strand exchange amplification
- LFA:
-
Lateral flow assay strip
- CS:
-
Complementary strand
- LOD:
-
Limit of detection
- UC:
-
Upconversion
- DC:
-
Downconversion
- PDT:
-
Photodynamic therapy
- ROS:
-
Reactive oxygen species
- AIE:
-
Aggregation-induced emission
- SMILES:
-
Small-molecule ionic isolation lattices
Nanomaterialien
- Sensoren und Prozessoren konvergieren für industrielle Anwendungen
- Was kommt als nächstes für den 3D-Druck – biomedizinische Anwendungen
- Die fünf wichtigsten Probleme und Herausforderungen für 5G
- Nanopartikel für die Krebstherapie:Aktuelle Fortschritte und Herausforderungen
- Graphen- und Polymerverbundstoffe für Superkondensatoranwendungen:ein Rückblick
- Neueste Fortschritte und Anwendungen in der IoT-Technologie
- EconCore lizenziert Fynotej für NA-Automobilanwendungen und macht Fortschritte bei Hochleistungsthermoplasten (HPT)
- Antriebe für industrielle Kälte- und industrielle Kühlanwendungen
- Schwenkdrehmaschinen für Bohr- und Drehanwendungen
- Die Vorteile und Herausforderungen der Hybridfertigung verstehen