2D-Halbleiter-Nanomaterialien und Heterostrukturen:Kontrollierte Synthese und funktionelle Anwendungen
Zusammenfassung
Zweidimensionale (2D) Halbleiter jenseits von Graphen stellen die dünnsten stabilen bekannten Nanomaterialien dar. Das schnelle Wachstum ihrer Familie und Anwendungen während des letzten Jahrzehnts des 21. Jahrhunderts hat den fortschrittlichen Nano- und optoelektronischen Technologien beispiellose Möglichkeiten eröffnet. In diesem Artikel geben wir einen Überblick über die neuesten Erkenntnisse zu den entwickelten 2D-Nanomaterialien. Fortschrittliche Synthesetechniken dieser 2D-Nanomaterialien und Heterostrukturen wurden zusammengefasst und ihre neuartigen Anwendungen diskutiert. Die Herstellungstechniken umfassen den Stand der Technik Entwicklungen der dampfphasenbasierten Abscheidungsverfahren und neuartige Van-der-Waals-(vdW)-Exfoliationsansätze zur Herstellung sowohl amorpher als auch kristalliner 2D-Nanomaterialien mit besonderem Fokus auf der chemischen Gasphasenabscheidung (CVD), Atomlagenabscheidung (ALD) von 2D-Halbleitern und deren Heterostrukturen sowie zur vdW-Exfoliation von 2D-Oberflächenoxidfilmen flüssiger Metalle.
Einführung
Nach dem Nobelpreis für Graphen (2010) und den nachfolgenden Fortschritten in der Entwicklung von 2D-Halbleitern über Graphen hinaus wurde im zweiten Jahrzehnt des 21. Jahrhundert [1,2,3,4]. In den Strukturen der berichteten 2D-Nanomaterialien wurden sehr interessante physikalisch-chemische Phänomene beobachtet, die dem Elektronentransfer und Bandlückenmodulationen zugeschrieben werden [5, 6]. Da Graphen keine Bandlücke hat, kann es keine Halbleitereigenschaften und enorme Aufmerksamkeit zeigen, und es wurden Anstrengungen unternommen, andere 2D-Nanomaterialien analog zu Graphen zu entwickeln, einschließlich Übergangsmetalldichalkogenide (TMDCs), hexagonales Bornitrid (h -BN), schwarzes Phosphen und Übergangsmetalloxide usw. [7,8,9,10,11,12,13,14,15,16]. Diese Familie von 2D-Nanomaterialien jenseits von Graphen zeigte ein breites Spektrum elektronischer Eigenschaften, das ein breites Eigenschaftsspektrum von Metallen über Halbmetalle und dann von Halbleitern bis hin zu Isolatoren abdeckte [17,18,19,20]. Neben dem breiten Spektrum elektronischer Eigenschaften orchestrieren andere Eigenschaften von 2D-Materialien, einschließlich ihrer großen Oberfläche, des Fehlens von baumelnden Bindungen, der Natur des Oberflächenzustands, der ausgezeichneten Spin-Orbital-Kopplungseigenschaften und ihrer Quantenspin-Hall-Effekte ziemlich faszinierende Eigenschaften in die einzelnen nanostrukturierten 2D-Materialien [21,22,23,24,25]. Folglich hat das Interesse der wissenschaftlichen Gemeinschaften an dieser Art von 2D-Nanomaterialien aufgrund ihrer beispiellosen Halbleitereigenschaften insbesondere im letzten Jahrzehnt exponentiell zugenommen. Dieses Interesse wurde wiederum durch den proportionalen exponentiellen Anstieg der veröffentlichten Artikel zu 2D-Halbleitern (Web of Knowledge™-Datenbank) in Abb. 1 angemessen widergespiegelt.
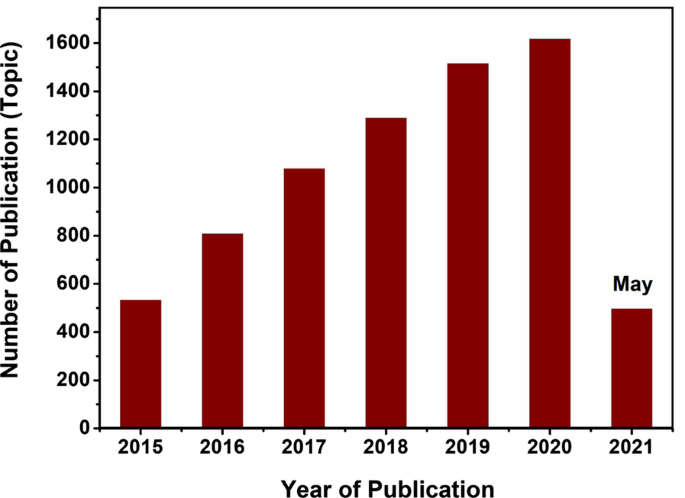
Dynamik der Anzahl veröffentlichter Artikel für „2D-Halbleiter ” basierend auf Web of Knowledge™
Die frühen Messungen der Eigenschaften von 2D-Halbleiter-Nanomaterialien zeigten einzigartige elektronische Eigenschaften, die nicht mit den Eigenschaften ihrer mikrostrukturierten und massiven Gegenstücke vergleichbar waren. Diese herausragenden Merkmale stehen im Zusammenhang mit den Quanten-Confinement-Effekten, die von der nanoskaligen Niedrigdimensionalität von 2D-Materialien herrühren [26]. Daher bieten diese einzigartigen Spezifikationen von 2D-Materialien ausgezeichnete Plattformen zum Studium ihrer grundlegenden Physik und Chemie. Die erste umfassend untersuchte Klasse von 2D-Nanomaterialien sind die geschichteten TMDCs (XY 2 , wobei X = Mo, Ti, W und Y = S, Se und Te) [27,28,29,30,31,32,33,34,35,36,37,38,39]. Die in dieser Phase erhaltenen Ergebnisse haben bestätigt, dass die Dichalkogenid-Halbleiter beträchtliche Unterschiede in der 2D- und Volumenleitfähigkeit aufweisen (σ 1 /σ 2 ~ 10 2 –10 3 ) [40]. Darüber hinaus können einige der einschichtigen 2D-Materialien wie Molybdändisulfid (MoS2 ), ist der Halbleiter mit direkter Bandlücke [41]. Die erhaltenen elektronischen Eigenschaften von 2D-TMDCs haben entscheidende Auswirkungen auf die Weiterentwicklung der nächsten Generation moderner nano- und optoelektronischer Geräte. Diese Befunde und andere Anisotropien ergeben sich aus dem Vorhandensein starker Zwischenschichten X –J Bindung, die im Gegensatz zu den schwachen vdW-Wechselwirkungen zwischen den Schichten steht [42]. Beispiel:TiS2 kristallisiert in einer hexagonalen Schichtstruktur, wobei eine hexagonal gepackte Schicht von Ti-Atomen zwischen zwei hexagonalen Schwefelschichten für jede Monoschicht eingebettet ist [43]. Diese spezifische geschichtete Anordnung von Atomen ermöglichte die vdW-Delamination geschichteter 2D-Nanostrukturen. Folglich konzentrierten sich die meisten der früheren Berichte auf die Eigenschaften von 2D-Materialien, die durch mechanische und vdW-Exfoliationsverfahren synthetisiert wurden. Aufgrund der ultradünnen Beschaffenheit von 2D-Folien können sie transparent, leicht und flexibel sein und können zudem sehr interessante Eigenschaften besitzen. Diese einzigartigen Eigenschaften ebneten den Weg für die Entwicklung von Hochleistungselektrodenmaterialien mit Anwendungen zur Energiespeicherung und -umwandlung, wie Batterien, Superkondensatoren und Brennstoffzellen [44]. Abbildung 2 fasst beispielsweise die Haupteigenschaften von 2D-Halbleitern und Heterostrukturen zusammen, die in ihren typischen Anwendungen genutzt wurden.
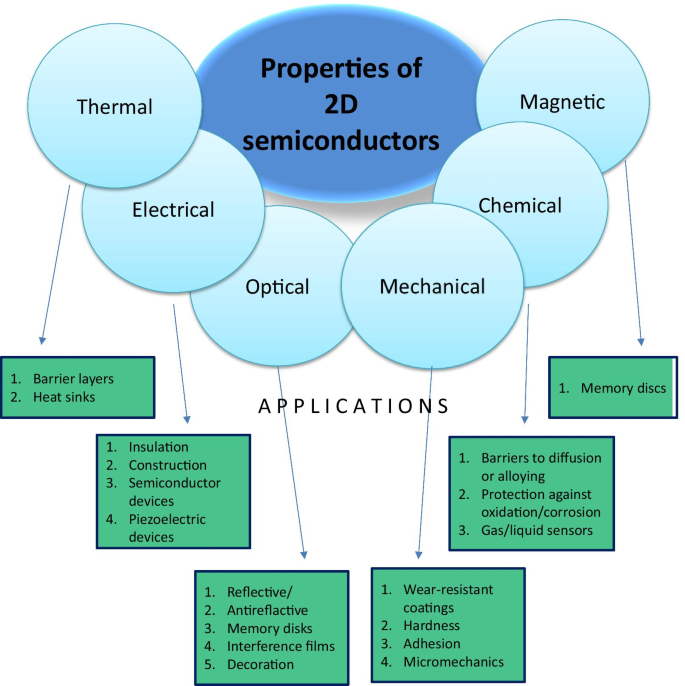
Eigenschaften von 2D-Halbleitern in ihren typischen Anwendungen
Bemerkenswert ist, dass sich das Gebiet der 2D-nanostrukturierten Halbleiter aufgrund der neuesten technologischen Fortschritte in der Nanofabrikation unabhängig als wichtiges Wissen für die Entwicklung moderner hochleistungsfähiger nano- und optoelektronischer Instrumente erweist [45]. Die Schichtstruktur von 2D-Nanomaterialien auf atomarer Ebene ist der Ursprung ihrer herausragenden Eigenschaften, wobei die genaue Abstimmbarkeit der Materialeigenschaften durch die Kontrolle ihrer Abmessungen auf atomarer Ebene bereitgestellt wird. Daher ist die konforme Synthese ultradünner 2D-Filme mit einheitlicher Dicke als grundlegender Schritt zur Entwicklung von Herstellungsverfahren fortschrittlicher elektronischer Instrumente auf Basis von 2D-Nanomaterialien äußerst wünschenswert. Insbesondere für die Herstellung ultradünner Schichten im Wafermaßstab mit präziser und gleichmäßiger Dicke über dem gewünschten Substrat müssen erfolgreiche Synthesetechniken zur Herstellung von Instrumenten auf Basis von 2D-Halbleitern entwickelt werden [46]. Darüber hinaus könnten 2D-Nanomaterialien während der Synthese- und Herstellungsphase dekoriert werden und in direktem Kontakt mit verschiedenen Komponenten elektronischer Einheiten stehen, einschließlich elektrischer Leiter, Isolatoren und Substrate mit verschiedenen geometrischen Merkmalen. Es sollte auch berücksichtigt werden, dass die Heterogrenzflächen zwischen 2D-Nanomaterialien und anderen Komponenten deren Eigenschaften grundlegend beeinflussen können. Dies hängt insbesondere mit den Ladungstransfermechanismen zwischen 2D-Schichten zusammen [47]. Die einzigartigen Eigenschaften von heterostrukturierten 2D-Filmen ermöglichen ihren Einsatz in vielen Anwendungen, einschließlich heterostrukturierter Elektronik, Optoelektronik, Biosensorik, Umweltsensoren, Katalyse, tragbarer und flexibler Elektronik, Memristoren und synaptischen Geräten [36, 44, 48 ,49,50,51,52,53,54,55]. Somit sollten die Synthese- und Herstellungstechniken wiederum auf die Herstellungskomplexität der funktionellen Instrumente auf Basis von 2D-Nanomaterialien reagieren. Darüber hinaus werden die elektronischen Eigenschaften von 2D-Materialien stark von den Materialeigenschaften beeinflusst, einschließlich der physikalischen und chemischen Strukturen, Abmessungen, Anzahl der Schichten, Morphologien, Orientierungen, Strukturphasen, Dotierungsdefekten, Amorphizität oder Kristallinität und den Korngrenzen in ihren Strukturen [56]. Die Anzahl der Schichten in 2D-Nanostrukturen hat einen zusätzlichen grundlegenden Einfluss auf die elektronische Struktur, und dann bestimmen die Eigenschaften von 2D-Materialien die endgültige Leistung der elektronischen Geräte basierend auf den 2D-Filmen [57].
Andererseits kann die Kristallinität oder Amorphie von 2D-Materialien auch ihre elektronischen Eigenschaften verändern. Abhängig von den Synthesemethoden und deren Bedingungen können 2D-Nanomaterialien entweder fehlerfrei . sein (Einkristall) oder enthalten ein gewisses Maß an Verunreinigungen oder wenige Korngrenzen. Daher wird die Ladungsmobilität durch das Phänomen der Grenzflächenstreuung über die Anzahl der Korngrenzen beeinflusst [58]. Während eine einkristalline Struktur die höchste Ladungsbeweglichkeit und geringe Grenzflächenstreuung aufweist, stellen die amorphen 2D-Strukturen die besten Isoliereigenschaften dar [58]. Die meisten der früheren Synthesemethoden von 2D-Nanomaterialien basierten auf der mechanischen Exfoliation von 2D-Nanostrukturen. Aufgrund schwacher vdW-Kräfte zwischen den geschichteten Strukturen kann die mechanische und chemische Exfoliation effektiv genutzt werden, um die geschichteten 2D-Nanostrukturen zu extrahieren [59]. Somit führt die mechanische Exfoliation zur Trennung der hochwertigen kristallinen 2D-Strukturen von ihren Wirtsmaterialien. Allerdings konnte die mechanische Exfoliation die großflächigen 2D-Nanofilme bereits nicht synthetisieren. Analog ist die Flüssigphasen-Exfoliation von 2D-Materialien ein weiterer geeigneter Ansatz für die kostengünstige Massenproduktion von 2D-Materialien. Dennoch sind die Qualitätskontrolle und die Größengleichmäßigkeit die Hauptherausforderungen für die Synthese von 2D-Filmen über diese Exfoliationstechnik [58]. Im Gegenteil, das direkte Wachstum von 2D-Filmen mittels dampfbasierter Abscheidungstechniken erwies sich als eine der zuverlässigsten und anwendbarsten Synthesemethoden zur Herstellung hochwertiger 2D-Nanofilme im Wafermaßstab mit präziser Dimensionskontrolle. CVD und ALD sind beispielsweise zwei wesentliche dampfbasierte Abscheidungstechniken, die unterscheidbare technische Vorteile für die großflächige Herstellung von nanostrukturierten 2D-Halbleitern und deren Heterostrukturen bieten [60, 61]. Die genaue Kontrolle der Dicke, der chemischen Zusammensetzung, des Kristallinitätszustands und sogar des Prozentsatzes an Dotierelementen und Strukturdefekten kann durch sorgfältige Manipulation der Wachstumsparameter in CVD- bzw. ALD-Prozessen angemessen erreicht werden. Abgesehen von den herkömmlichen Methoden zur Synthese von 2D-Filmen sind vor wenigen Jahren auch andere neue Quellen für 2D-Materialien aufgetaucht. Beispielsweise ist das Oberflächenoxid flüssiger Metalle in der Tat eine einzigartige Quelle für hochwertige natürliche 2D-Nanofilme mit beispiellosen Eigenschaften, die sich aus den physikalischen und chemischen Eigenschaften flüssiger Legierungen ergeben [61].
Daher werden in der Übersicht die neuesten Erkenntnisse zu den neuartigen Synthesemethoden für 2D-Nanomaterialien diskutiert, wobei der Schwerpunkt auf ALD- und CVD-Techniken sowie auf den vdW-Exfoliationen des 2D-Oberflächenoxids flüssiger Metalle liegt. Die Wachstumsmechanismen von 2D-Materialien in der Gasphasenabscheidung werden erläutert und die wichtigsten Herausforderungen und Chancen bei der Herstellung und Abscheidung von 2D-basierten Halbleitermaterialien werden ebenfalls diskutiert. Darüber hinaus wird die kürzlich entwickelte vdW-Exfoliationstechnik der 2D-Oxidfilme auf natürlichen Oberflächen flüssiger Metalle vorgestellt. Endlich der Stand der Technik entwickelte funktionale Anwendungen ultradünner 2D-basierter Bauelemente einschließlich ihrer elektronischen und optoelektronischen Eigenschaften werden vorgestellt und detailliert charakterisiert.
2D-Nanomaterialien, synthetisiert durch dampfbasierte Herstellungstechniken
Die Forschungsschwerpunkte im Bereich 2D-Halbleitermaterialien liegen in der Synthese und anschließenden Charakterisierung von 2D-Nanostrukturen. Diese 2D-Nanostrukturen gehören zu einer breiten Palette von anorganischen, organischen und polymeren Materialien mit atomarer und dünner Dicke. Die Synthesestrategien folgen normalerweise zwei Hauptansätzen, d. h. dem top-down und von unten nach oben Strategien. Daher spielt die Entwicklung von 2D-Halbleitern mit kontrollierten Architekturen und physikalischen und chemischen Eigenschaften in verschiedenen Anwendungen eine Schlüsselrolle und wird neue Möglichkeiten auf einem Niveau eröffnen, das deutlich über den heutigen kommerziellen Halbleitertechnologien liegt. Trotz erheblicher Anstrengungen und einzigartiger wissenschaftlicher Entdeckungen bei den 2D-Halbleitern in den letzten Jahren wurden die meisten von ihnen jedoch in den Labors mit recht einfachen Exfoliationsverfahren gewonnen, die in industriellen Anwendungen nicht verwendet werden können. Eine Alternative stellt das Top-Down dar Methode, die typischerweise die Delaminierung und vdW-Exfoliation von geschichteten Materialien und geschichteten kovalenten organischen Gerüsten (COFs) beinhaltet. Der Flaschenhals dieses Verfahrens ist jedoch die extrem geringe Produktausbeute und das Umstapeln der Platten während der Erstarrung. Eine andere Alternative basiert auf dem bottom-up anisotrope Anordnung von anorganischen, organischen oder polymeren Vorstufen in 2D-Weise. Trotz einiger Erfolge bei der Herstellung von 2D-Nanomaterialien bleiben die eingehenden Untersuchungen von rational gestalteten 2D-Nanoblättern mit verschiedenen Funktionalitäten in der elektrochemischen Energiespeicherung und -umwandlung primitiv. Dabei wird die vollständige Erforschung der Einzigartigkeit von 2D-Nanomaterialien mit maßgeschneiderter Dicke, großer Oberfläche, guter Leitfähigkeit und mechanischer Flexibilität zur Beschleunigung der Ionendiffusion und des Elektronentransports in verschiedenen Anwendungen stark nachgefragt. Die folgenden Abschnitte geben einen Überblick über die Grundlagen von zwei Hauptabscheidungstechniken von 2D-Materialien, d. h. CVD und ALD mit Fokus auf die Errungenschaften der letzten Jahre.
CVD von 2D-Nanostrukturen
Das dampfphasenbasierte Direktwachstum ultradünner Filme gehört zu den zuverlässigsten und anwendbarsten Syntheseverfahren zur Abscheidung hochwertiger Filme mit ultrapräzisen Maßangaben [62]. Unter verschiedenen Methoden stellt CVD die erste bekannte erfolgreiche Technik zum Aufwachsen ultradünner 2D-Filme auf mehreren unterschiedlichen Substraten dar. CVD bietet einen skalierbaren und kontrollierbaren Ansatz für das Wachstum hochwertiger großflächiger 2D-Filme [63]. Es gibt mehrere herausragende Spezifikationen, die das CVD-Verfahren zu einer zuverlässigen Technik für die kontrollierbare Abscheidung von 2D-Nanomaterialien machen. Das Verfahren basiert auf der schrittweisen Reaktion gasförmiger Stoffe im Dampfzustand auf der Oberfläche von Substraten. Auf die CVD-Reaktionen folgt das Wachstum von Festkörper-Dünnfilmen mit wenigen Atomlagendicken. Daher hängen die Eigenschaften von 2D-Nanomaterialien stark von den Grenzflächeneigenschaften, den geometrischen Eigenschaften des Substrats und den strukturellen Phasen von Festkörperfilmen ab. Diese Eigenschaften konnten durch die genaue Einstellung der CVD-Wachstumsparameter sorgfältig moduliert werden. Um die allgemeinen Mechanismen des CVD-Wachstums zu verstehen, sollte erkannt werden, wie die CVD-Parameter einschließlich Temperatur, Druck, Substrat und Vorläufer den Stoff-, Wärme- und Grenzflächenreaktionen an der Oberfläche beeinflussen können. In dieser Hinsicht muss eine gut durchdachte Rezeptur entwickelt werden, die die Hauptabscheidungsparameter während des Prozesses steuert [64]. Darüber hinaus ermöglicht es die Kontrolle des Dotierungsniveaus in 2D-Nanofilmen, was eine entscheidende Fähigkeit beim Design von Halbleiter-Nanostrukturen mit spezifischen Eigenschaften ist [64]. So ist es beispielsweise möglich, den Gehalt an Chalkogen und Metallleerstellen während des CVD-Prozesses zu kontrollieren. Die folgenden Schlüsselparameter des CVD-Prozesses hängen stark von den strukturellen Phasen, der Morphologie und den Grenzflächenreaktionen ab.
Temperatur
Die Temperatur ist einer der wichtigsten fundamentalen Parameter, die mehrere miteinander verbundene Faktoren während des CVD-Filmwachstums direkt beeinflussen, einschließlich der Geschwindigkeit chemischer Reaktionen von Vorläufern, der Flussrate des Trägergases und schließlich der Beeinflussung der Wachstumsrate des CVD-Films [64]. Im Allgemeinen gewährleistet eine hohe CVD-Temperatur die Abscheidung der hochwertigen 2D-CVD-Filme. Allerdings hat eine extrem hohe Temperatur auch Nachteile. Insbesondere kann es hohe Konzentrationsgradienten erzeugen und verursacht daher einen instabilen Massenfluss und Transfer im System [64]. Es beeinflusst auch direkt den Sättigungsdruck der Komponenten in der Kammer und verändert folglich die Wachstumsrate von Dünnschichten. Die kombinierten Auswirkungen von Temperatur und Druck können während der CVD-Abscheidung von 2D-TMDC-Filmen beobachtet werden. Bei dieser Art von 2D-Nanostrukturen ist die Konzentration von Schwefel- und Selenelementen im System sehr wichtig, um die endgültige Zusammensetzung von 2D-Filmen zu bestimmen. Hoher Gasdruck und hohe Konzentration ermöglichten beispielsweise die kontrollierbare Synthese von 2D-TMDCs (MoS2 , WS2 , etc.). Im Allgemeinen erleichtert eine hohe CVD-Temperatur, begleitet von einem ausreichenden Druck, den thermodynamisch aktivierten Abscheidungsmechanismus, während eine niedrige CVD-Temperatur normalerweise zum kinetischen Wachstumsprozess führt [64, 65]. Der kontrollierte Wachstumsmechanismus führt schließlich zum kontrollierten Wachstum von 2D-TMDCs mit der Möglichkeit zur Anpassung der Eigenschaften und einer Reihe von fundamentalen Schichten.
Druck
CVD ist auch als Abscheidungstechnik mit variablem Druck bekannt, bei der der Prozessdruck von Atmosphärenniveau auf wenige Millimeter geändert werden kann. Die günstigste Voraussetzung für einen kontrollierbaren CVD-Prozess wird bei der geringen Konzentration und hohen Geschwindigkeit der Massenzufuhr erreicht. In dieser Hinsicht ist der niedrige Druck immer zuverlässiger für ein gleichmäßiges Wachstum ultradünner 2D-Nanostrukturen bei der Abscheidung von TMDCs 2D-Nanostrukturen im Wafer-Maßstab [66, 67]. Der Partialdruck von Komponenten kann auch das gleichmäßige Schicht-für-Schicht-Wachstum von 2D-TMDCs-Filmen direkt beeinflussen. Daher ist das Wachstum von 2D-MoS2 Film durch CVD-Technik ist eines der bekanntesten Beispiele, bei dem die Herstellung der zweiten Grundschicht über dem anfänglich gewachsenen Film nur an den Korngrenzen der ersten abgeschiedenen Schicht bei niedrigem Druck begonnen werden kann.
Substrat
Die Beschaffenheit des Substrats hat grundlegende Auswirkungen auf das Wachstum von 2D-Filmen und Heterostrukturen. Zum Beispiel Metallsubstrat, Si, SiO2 , Glimmer und Polyimide gehören zu den am häufigsten verwendeten Substraten für die CVD-Abscheidung von 2D-Filmen [68, 69]. Die Rolle der Substrate beim Wachstumsmechanismus von 2D-Filmen bestimmt deren Kristallinität, Kornorientierung, Mikrostrukturen und Eigenschaften. Insbesondere 2D-Metalloxidfilme, die auf Gold-, Nickel-, Kupfer- und Silbersubstraten abgeschieden wurden, zeigten die katalytisch aktiven Eigenschaften [70,71,72]. Im Allgemeinen hängt bei metallischen Substraten das bevorzugte Wachstum von 2D-Filmen mit spezifischen Kristallrichtungen technisch von den flächenabhängigen Bindungsenergien zwischen dem metallischen Substrat und dem abgeschiedenen TDMC-Film ab [73]. Es beeinflusst auch direkt die Morphologie von gewachsenen Nanostrukturen. Im Fall von 2D-Metalloxiden, die auf einem Au-Film abgeschieden werden, bestimmen die Bindungsenergien zwischen Au und Sauerstoff (O) und Au und Metall (M) des 2D-Oxidfilms die chemischen Bindungsstrukturen an der Heterogrenzfläche zwischen Au und Metalloxidfilm [73]. Die bevorzugte Bindung zwischen Au und Metallatomen des Oxidfilms wird vorhergesagt, was hauptsächlich zur Bildung von Au-M-O-Grenzflächen führt [74,75,76]. Die starke Wechselwirkung zwischen Au oder anderen metallischen Substraten und abgeschiedenen Oxiddünnschichten ermöglichte das adaptive Wachstum von 2D-Oxiden aus der kristallinen Struktur von Metallsubstraten [77,78,79]. Dies bedeutet, dass das Wachstumsmuster der ersten Metalloxid-Monoschicht dem kristallinen Muster des metallischen Substrats folgt. Während beispielsweise das Au die kubisch-flächenzentrierte (fcc) Kristallstruktur hat, bestimmt seine Kristallrichtung auf der Oberfläche des Au-Substrats die Kristallorientierung des 2D-Metalloxidfilms. Bemerkenswert ist, dass das unkonstruierte Au (111) aus hexagonalen Gittern besteht, während eine rekonstruierte Au (111)-Ebene eine komplexe Struktur aufweist [80, 81]. Es wurde beobachtet, dass verschiedene abgeschiedene Oxidfilme auf dem Au-Substrat die Fischgrätrekonstruktion anheben, die durch die starke Wechselwirkung zwischen Au (111)-Facette und dem ultradünnen Oxidfilm verursacht wurde. Auf den Au-Substraten wurden mehrere verschiedene 2D-Metalloxide aufgewachsen, darunter TiOx , VOx , CoO, MoO3 , MgO, ZnO und WOx [82]. Eines der bekanntesten Beispiele für Katalysatoren ist 2D TiO2 auf der Oberfläche des Au-(111)-Substrats aufgewachsen [83]. Es wurde vorgeschlagen, dass Ti-Atome die dreifachen Hohlstellen des Au-Gitters und O-Atome an den Brückenstellen der Ti-Atome besetzen, dann eine Wabenstruktur mit der Stöchiometrie von Ti2 O3 gebildet wird, dargestellt in Abb. 3a [83]. Darüber hinaus gibt es einen weiteren Wachstumsmechanismus für 2D-Oxidfilme, bei dem ein M-O-Gitter die Au (111)-Oberfläche überlagert und Moiré . bildet Muster, die zu unterschiedlich aussehenden Windradstrukturen mit TiO-Stöchiometrie führen, wie in Abb. 3b bzw. c gezeigt [83]. Das sechseckige Moiré Musterwachstum wurde häufig während der CVD von TiO, FeO [84], CoO [85] und ZnO [86] ultradünnen Filmen auf dem Au (111)-Substrat beobachtet.
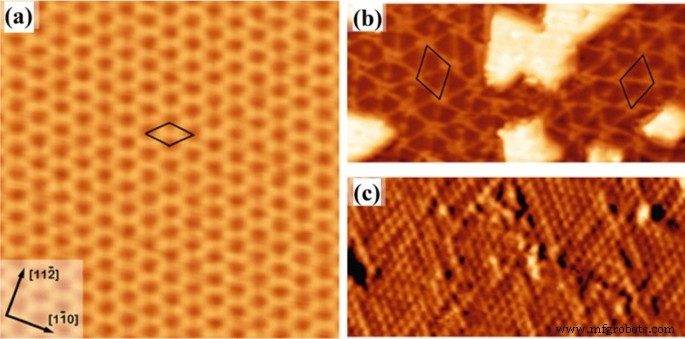
STM-Bild von a die Wabe Ti2 O3 Struktur und b auf Au (111) gewachsene Pinwheel-TiO-Monoschicht und c atomar aufgelöstes STM-Bild von Windradstrukturen auf Au (111). Reproduziert mit Genehmigung von [83]
MoS2 ist ein typischer Vertreter der Familie der 2D-TMDCS. Bisher gilt es als eines der günstigsten Nanomaterialien unter allen TMDCS, da es eine relativ kleine Bandlücke von 1,29 eV bzw. 1,90 eV für das Volumenmaterial bzw. die Einzelschichten aufweist [40, 87, 88]. Früher ein einkristallines MoS2 2D-Film wurde nur mechanisch von seiner Wirtsmasse abgeschält. Da es sich um einen Halbleiter mit direkter Gap handelt, sind einzelne Schichten aus MoS2 bieten die faszinierende Möglichkeit zur Realisierung eines Interband-Tunnel-Feldeffekttransistors (FET), der sich durch ein schärferes Einschalten als die theoretische Grenze von 60 mV dec −1 . auszeichnet für klassische Transistoren und folglich geringere Verlustleistung. Im Fall von Silizium, einem Halbleiter mit indirekter Lücke, ist diese Eigenschaft nach wie vor schwierig zu erreichen, da dort Zwischenbandübergänge Phononen und Rekombinationszentren erfordern [87]. Eine der interessanten neueren Anwendungen von 2D MoS2 Nanokristalle sind der Transistor [87] und der Phototransistor [40] basierend auf einer einzelnen Schicht von MoS2 Nanoblatt. Darüber hinaus wurde die erfolgreiche großflächige Abscheidung von 2D-Filmen durch modifizierte CVD-Techniken erreicht, darunter die metall-organische chemische Gasphasenabscheidung (MOCVD), die chemische Niederdruck-Gasphasenabscheidung (LPCVD), die chemische Gasphasenabscheidung mit induktiv gekoppeltem Plasma (ICP-CVD .). ) oder andere innovative Methoden, die mit anderen Techniken entwickelt und kombiniert werden. CVD ist auch für seine Fähigkeit zur Abscheidung von 2D-TMDC-Filmen im Wafermaßstab bekannt. Abbildung 4 zeigt eines der interessanten Beispiele, die Ablagerung von ultradünnem 2D-MoS2 . in größeren Bereichen und WS2 Filme über 4-Zoll-Wafer [88]. In diesem CVD-Prozess wird Mo(CO)6 und W(CO)6 und (C2 H5 )2 S wurden als Vorläufer in der MOCVD-Technik verwendet, während H2 /Ar wurde als Trägergas (Abb. 4a, b) im MOCVD-Prozess verwendet [88]. Es wurde gezeigt, dass 8100 Hochleistungs-FET-Einheiten auf Basis von 2D-MoS2 Filme wurden unter Verwendung einer Photolithographie-Technik mit dem gleichen Back-Gate-Substrat hergestellt (Fig. 4c). In der zweiten Konfiguration des Gerätes externes SiO2 Auf dem 2D-MoS2 . wurde ein dielektrischer Film abgeschieden Film zur Herstellung von FET-Instrumenten (Abb. 4d). Die elektrischen Messungen von Transistoren bestätigten die konsistente Leistung von FET, die aus den verschiedenen Waferteilen ausgewählt wurden. Daher die gleichmäßigen und konformen Wachstumsbedingungen während des CVD-Prozesses von 2D MoS2 und WS2 Filme wurden bestätigt (Abb. 4f).
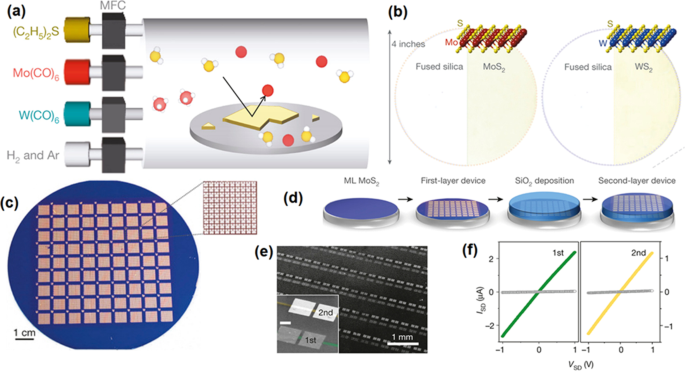
MOCVD-Wachstum im Wafer-Maßstab von kontinuierlichem MoS2 und WS2 Film. a Das grafische Schema des CVD-Geräts und der zugehörigen Vorläufer für MOCVD von 2D-MoS2 und WS2 Filme. c Die Charge von 8100 FET-Bauelementen basierend auf 2D-MoS2 Film über Si/SiO2 Waffel. Der Einschub zeigt das vergrößerte Bild eines Quadrats von 1 mm mit 100 Geräten. d Die sequentielle Fertigungsphase von gestapeltem MoS2 -basiertes Gerät. e Das Bild zeigt das MoS2 FET-Bauelemente auf ersten und zweiten Schichten. f Die IDS-VDS zweier benachbarter FET-Bauelemente auf zwei verschiedenen Schichten. Nachdruck mit Genehmigung von [88]
CVD hat sich auch als vielseitige Technik zur Herstellung verschiedener 2D-Heterostrukturen etabliert [89]. CVD wurde zum Beispiel für die erfolgreiche Abscheidung sowohl von seitlichen als auch von vertikalen Konfigurationen verwendet. Beim lateralen Stil werden die 2D-Nanofilme auf atomarer Ebene aneinander befestigt, während bei der vertikalen Struktur die Wechselwirkungskraft zwischen den Schichten vdW-Natur hat. Daher ist in den lateralen 2D-Heterostrukturen eine strikte Gitteranpassung für die Entwicklung der 2D-Hetero-Schnittstellen dringend erforderlich, wie für MoS2 . berichtet wurde /WS2 Heterostrukturen [89]. Einfache Abscheidung von MoS2 . in einem Schritt /WS2 laterale 2D-Heterostrukturen wurden aufgebaut, wenn die Vorstufen Schwefel, Molybdäntrioxid und Wolfram während des CVD-Prozesses verwendet werden (Abb. 5a, b) [89]. Die entwickelten 2D-Filme hatten scharfe atomare Heterogrenzflächen, wobei die Atomebene dieselbe kristalline Orientierung wie die Sessel- und Zickzack-Orientierung teilte (Abb. 5c). Die endgültige Morphologie des Films war eine dreieckige Kern-Schale-Form mit MoS2 innen und WS2 außen (Abb. 5d). Raman-Charakterisierungsstudien bestätigten die Herstellung sowohl vertikaler als auch lateraler 2D-Filme (Abb. 5f). Entwicklung p-n Heteroübergang zwischen 2D WS2 und MoS2 film hat den photovoltaischen Effekt von heterostrukturierten 2D-Filmen verstärkt. Daher wurde ein starkes Photolumineszenz-Phänomen nachgewiesen (Abb. 5e, g) [89]. Eine ziemlich scharfe Grenzfläche mit derselben kristallinen Orientierung und Gitterstruktur ähnlich wie bei WS2 und MoS2 2D-Filme wurde charakterisiert. Darüber hinaus wurde in einem anderen Bericht CVD verwendet, um das laterale 1H MoS2 . zu entwickeln /1T′ MoTe2 Hetero-Schnittstellen, bei denen die beiden Komponenten relativ große Gitterfehlanpassungen aufwiesen [90]. Es gibt mehrere andere Fälle, die die Entwicklung von Heterogrenzflächen zwischen 2D-TMDC-Filmen mit großer Gitterfehlanpassung demonstrierten, einschließlich 2D-WSe2 /MoSe2 [91] und MoS2 /WS2 [92] bestätigt die Fähigkeit des CVD-Verfahrens zur Abscheidung von lateralen 2D-Heterostrukturen von Halbleitern/Halbleitern. Daraus kann geschlossen werden, dass die moderne CVD-Technik ihre vielseitigen Fähigkeiten zur erfolgreichen Abscheidung von 2D-Materialien auf organischen und polymeren flexiblen Substraten, Isolatoren und Graphen-Substraten unter Beweis gestellt hat. Tabelle 1 fasst die neuesten Errungenschaften von 2D-TMDCs und ihren Heterostrukturen zusammen, die durch CVD-Technik im Wafermaßstab hergestellt wurden [93].
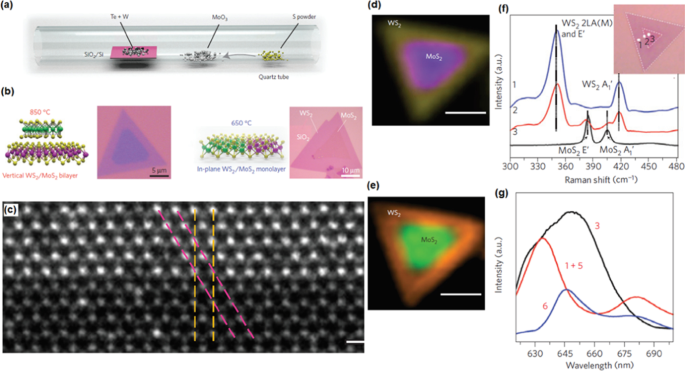
a Schema des CVD-Wachstums von lateralen 2D-Heterostrukturen von Halbleitern/Halbleitern. b STEM-Bilder mit atomarer Auflösung des Kontrasts der Grenzfläche zwischen WS2 und MoS2 . c Die Raman-Intensitätskartierung eines 2D-heterostrukturierten 2D-Films bei 351 cm −1 (gelb) und 381 cm −1 (Violett). d Die kombinierte PL-Intensitätskartierung bei 630 nm (orange) und 680 nm (grün). Nachgedruckt und reproduziert mit Genehmigung von [89]
Im Allgemeinen ist die Stabilität der CVD-hergestellten 2D-Nanomaterialien und Heterostrukturen recht hoch, obwohl die Informationen über die Langzeitstabilität solcher Nanostrukturen in den meisten veröffentlichten Arbeiten eher begrenzt sind. Die Langzeitleistung und Stabilität der CVD-gezüchteten TMDC-basierten elektronischen und optoelektronischen Instrumente wird stark durch die Grenzflächen, Defekte und Korngrenzen in den Nanomaterialien beeinflusst, da sie als Trägerstreuzentren dienen. Dies verringert wiederum die Trägermobilität der Geräte. Daher sollte die Herstellung von 2D-Verbundmaterialien mit sauberen Oberflächen, hoher Qualität und Domänengröße im Wafer-Maßstab ein angestrebtes Ziel für zukünftige Nano- und Optoelektronik sein. Es sollte auch betont werden, dass eine große Anzahl anderer 2D-Verbindungsmaterialien noch nicht gut untersucht oder erfolgreich gezüchtet wurden und folglich weitere spannende Entdeckungen darauf warten, entdeckt zu werden.
ALD von 2D-Nanostrukturen
ALD als Modifikation von CVD kann verschiedene Strategien zur dickenkontrollierten Herstellung von 2D-Halbleitern und Sandwich-Heterostrukturen implementieren. Obwohl ALD ursprünglich vor etwa 45 Jahren entwickelt wurde, gewinnt es erst zu Beginn des 21. Jahrhunderts aufgrund großer Vorteile und Entwicklung verschiedener Vorläufer [94] an Popularität. Während des letzten Jahrzehnts wurde es zur Herstellung einer Vielzahl von Materialien verwendet, darunter Oxide, Sulfide, Selenide, Telluride, Nitride und Metalle [95]. Eine wesentliche Anforderung besteht darin, dass geeignete molekulare Vorstufen selbstlimitierende Reaktionen eingehen müssen, da der Hauptunterschied zwischen der ALD und anderen Dampfabscheidungsverfahren darin besteht, dass nach jeder Vorstufenbelichtung eine selbstsättigende Oberflächenmonoschicht gebildet wird. Eine vollständige Beschreibung der neuesten Entwicklungen in der ALD-Chemie findet sich in aktuellen Übersichtsartikeln [94,95,96]. Thus, so far ALD has been implemented in a diverse number of fields including microelectronics [97], catalysis [98], photovoltaics [99] and sensors [100]. Unfortunately, the ALD implementation toward the synthesis of 2D semiconductors and 2D sandwich nanostructures is rather limited.
As deposition technique, ALD consists of a series of self-limiting, surface-saturated reactions to form thin conformal films at a controllable rate. Despite all obvious ALD advantages, it has to be admitted that this emerging technology has not yet been fully exploited for fabrication of different 2D nanostructures. Apparently, the lack of reliable recipes and precursors, specific ALD temperature window for deposition are some of the valid reasons for that. On the other hand, it was recently reported that the ALD advantages are far superior to the existing capabilities of other deposition techniques [101]. Specifically, ALD is the only one technology, which enables fabrication of defects-free, conformal 2D nanofilms and their heterostructures on the wafer scale with precise, Ångstrom scale control of their thickness during deposition [102]. In this regard, the state-of-the-art nanoscale ALD interfacing and molecular engineering of 2D nanomaterials can open up completely new possibilities by providing ultra-thin channels for key doping, minimization of the density of interfacial impurities and optimization of capabilities of instruments. Furthermore, the ALD fabrication technique enables turning the design of 2D nanostructures toward heterojunctions and hetero-structures [103,104,105], and hybrid SE based on inorganic–inorganic [106], organic–organic and inorganic–organic nanomaterials [107]. Figure 6 illustrates growing interests to ALD as well as comparison of the deposition rates and properties for 2D nanostructures developed by various deposition techniques [94].
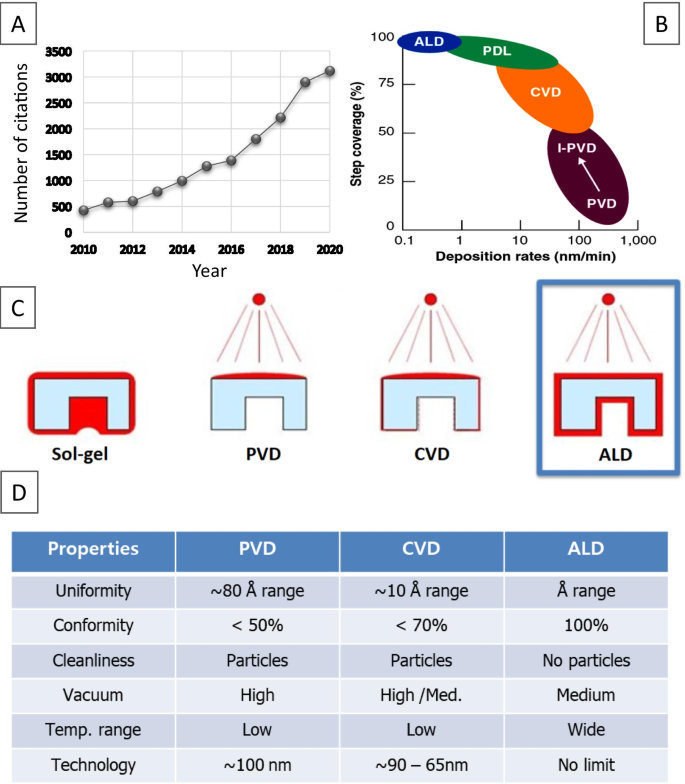
Number of annual citations on “Atomic layer deposition” topic a of published manuscripts. Data are according to Web of Knowledge™ Database. Comparison of the deposition rate vs step coverage (b ) and coverage areas produced by different deposition techniques (c ). Comparative analysis of properties of SE fabricated by PVD, CVD, and ALD, respectively (d ). Reproduced with permission from [94]
ALD of 2D Metal Chalcogenide Films
The layered TMDCs are composed of sandwiched structure where the metal layers covalently bonded with two individual surrounding chalcogen atoms, thus the layer-by-layer deposition mechanism in ALD technique is favourable for making layered 2D TMDC nanostructures. Among the different 2D TMDCs films including binary, ternary and doped chalcogenides, less than 20 type of them could be deposited via ALD method. The first MoS2 nanofilm was fabricated by ALD in 2015. Initially, the number of MoS2 layers was controlled by the tuning of parameters of ALD technique [106, 108,109,110]. Later, the ALD synthesis of TMDCs monolayer was reported [111,112,113,114]. The self-limiting layer synthesis (SLS) of MoS2 monolayer film was also facilitated based on the typical ALD half-reactions [115, 116]. Here, the some practical cases of ALD of stable 2D films of metal dichalcogenides are summarized in Table 2 [94].
The first investigation of 2D MoS2 deposition was based on the sulfurization of ALD deposited MoOx few-layered film. The formation of S–Mo-S arrangements of atoms along the z -axis was achieved after 10 min interaction of MoOx film with S gas at 600 °C [117]. The morphology, uniformity, crystallinity, nucleation and the quality of ALD-deposited film have direct impact on the final properties and characteristics of the MoS2 film [117,118,119,120,121]. In this example, the parameters of multistep annealing process should be controlled precisely to facilitate the formation of MoS2 films with sequential reduction of Mo and then incorporation of S atoms. In addition, the multistep reactions allowed the coverage control and phase transformation from crystalline MoS2 to the 2H-phase at the higher post-treatment temperatures. The first wafer-scale CVD of MoS2 on the 2-inch sapphire substrate was reported in 2014, when MoCl5 und H2 S were employed as the precursors in the ALD system at 300 °C [121]. It was found that 10 sequential ALD cycles are required to establish the uniform growth of the MoS2 film and prevent the islands growth. The following post-annealing of ALD MoS2 film at 800 °C improved the crystallinity of MoS2 at the expense of losing the thickness uniformity of MoS2 on the wafer substrates. Thus, higher number of ALD cycles were done (20 cycles) to compensate the lack of uniformity due to the high-temperature post-annealing process. Considering the ALD window, it was found that the ALD process within 350 to 450 °C permits higher quality of sulfide layers with the growth rate of 0.87 Å/cycles [122]. It was also demonstrated that the following annealing of as-deposited MoS2 film in H2 S or sulfur gas can improve the stoichiometry of 2D MoSx film [123].
The deposition of 2D MoS2 films via ALD technique can also be achieved by usage of other precursors. Other main precursors for deposition of MoS2 2D films were Mo(CO)6 , H2 S and dimethyl disulfide compounds. The ALD window suitable for these precursors is in the range of 155–170 °C. By monitoring the mass gain during the ALD cycles, it was revealed that multiple pulses of Mo source are required to reach the saturation, while a single H2 S injection is sufficient to saturate the reaction and achieve the growth rate of 2.5 Å/cycle in the optimized condition [124]. The plasma-enhanced atomic layer deposition (PE-ALD) can also be successfully employed to deposit ultra-thin h -MoS2 film on the different substrates. The growth rate of 0.5 Å/cycle was observed at the ALD window of (175–200 °C) for deposition of 2D MoS2 Filme. Lower ALD window (60–120 °C) caused the deposition of amorphous 2D MoS2 Filme. Particularly, the ALD at 100 °C allowed the formation of continuous layer of amorphous MoS2 on the Si wafer. To improve the crystallinity of as-deposited ALD film, the later rapid thermal annealing (RTA) treatment at 900 °C led to the formation of crystalline hexagonal layer of MoS2 [125, 126]. Another recipe for deposition of 2D MoS2 film is Tetrakis (dimethylamido) molybdenum (Mo(NMe2 )4 ), which interacts with either H2 S or alternatively 1, 2 ethanedithiol (HS(CH2 )2 SH) to deposit 2D MoS2 Filme. In this recipe, the ALD of MoS2 film taken place at the lower temperature (T < 120 °C) since the Mo(NMe2 )4 decomposes at temperature higher than 120 °C. The as-deposited 2D film was amorphous in this technique. Thus, the subsequent annealing was employed to crystalize the 2D MoS2 Filme. The mass spectroscopy analysis revealed that the mechanism of ALD deposition of MoS2 film is based on the removal of the remaining NMe2 ligands from the substrate surface after interaction of sulfur gas with Mo precursor. It was roved that it is possible to deposited 2D films on polymeric substrates by using lower ALD temperature.
2D WS2 is another example of the layered dichalcogenide ALD deposited at 300 °C by using WF6 und H2 S as precursors [127]. A Zn-based catalyst is required to initiate the deposition of WS2 film via ALD technique. Diethylzinc (DEZ) and H2 S were employed to assist the rapid nucleation of WS2 film over a wide range of substrates [128]. Zn acts as reducing agent for WF6 favouring the adsorption of WF6 on the surface of substrates. The initial growth rate of 1.0 Å/cycle was recorded, which then was reduced after 50 ALD cycles of WS2 Ablage. It was found that the deposition of a monolayer ZnS film is also facilitated the growth of WS2 film with crystalline structure [129, 130]
WSe2 is another TMDC 2D film which was fabricated by ALD [124]. In this report, a self-limiting approach for WCl6 and diethyl selenide (DESe) at the higher ALD temperature of 600–800 °C was employed and the dependence of film thickness on the ALD processing temperature was observed [131]. Five, three and one crystalized layers of WSe2 were deposited on the Si/SiO2 wafers (8 cm 2 ) at 600 °C, 700 °C and 800 °C, respectively. After 100 ALD cycles, the growth of WSe2 was stopped due to growth saturation. The deposited WSe2 film with few layers and monolayer was crystallined with honeycomb structure. The same film was employed as a p -type semiconductor in the FET and showed the capability of ALD deposited WSe2 film for application in electronic devices. In another parallel attempt, the thermal ALD of continues WSe2 film on the Si/SiO2 substrate was successfully carried out at 390 °C [132]. WCl5 und H2 Se were employed to deposit a highly uniform film with well-crystalline structure. The quality and crystallinity of the ALD WSe2 film were fairly compatible to the mechanically exfoliated and CVD WSe2 films [132].
SnSx 2D family is the other types of 2D materials which was extensively studied during last few years. The first deposited SnS film was reported by using tin (II) 2,4-pentanedionate (Sn(acac)2 ) precursor as the Sn source and H2 S as the sulfur source, respectively, where the growth rate of 0.23 Å/cycle was achieved in the ALD window from 125 to 225 °C. The other precursors for ALD of SnS film are bis(N ,N ’-diisopropylacetamidinato) tin (II) (Sn(amd)2 ) and N2 ,N3 -di-tert-butylbutane-2,3-diamine tin (II) which react with H2 S gas during ALD [133]. This recipe enabled the deposition of crystalline SnS film at the ALD temperature of less than 200 °C.
2D-SnS2 and SnSx films are deposited via employment of precursors containing Sn atoms [134]. The tetrakis (dimethylamino) tin (TDMASn) [135, 136] and tin acetate (Sn(OAc)4 ) [137] as the Sn (IV) metal precursors were used for ALD of SnS2 Filme. Polycrystalline hexagonal SnS2 films were deposited with the growth rate of 0.64–0.8 Å/cycle [134] at the temperature range of 140–150 °C. The growth of ALD temperature above 160 °C resulted in the development of thin mono-sulfide films. However, the as-deposited film always contains a combination of both mixed SnS and SnS2 Verbindungen. Crystallinity of the film can still be improved by the following post-treatment annealing in H2 S gas at 300 °C. As an example, the formation of a single phased orthorhombic 2D SnS2 films with interlayer spacing of 0.6 nm was reported after the post-annealing treatment at 300 °C in H2 Atmosphäre. Another precursor for ALD deposition of SnS2 film is Sn(OAc)4 where the ALD reaction in the ALD temperature of 150 °C resulted in the growth rate of 0.17 Å/cycle for the amorphous stoichiometric SnS2 Film. Generally, in ALD deposition of few-layered SnSx films with Sn precursors and H2 S gas, the chemical composition, structure and the stoichiometry of SnSx ALD structures can be modulated depending on the deposition conditions and the post-ALD treatments [137].
One of the main ALD capabilities is the wafer-scale deposition of 2D hetero-structured TMDCs, which opens up further opportunity for fabrication of vdW hetero-structures. The technological challenges for deposition of 2D hetero-structured films are the main concerns for their deposition. Basal plans of the most of 2D materials are inert with the lack of dangling band, thus the nucleation of 2nd family of 2D films over the first layer is considerably challenging. Therefore, it is necessary to enable the initial adsorption of precursors on the surface of 2D film and then facilitate the growth of second 2D film over substrate. One of such examples is the ALD deposition of Sb2 Te3 film on the surface of graphene by using SbCl3 and (Me3 Si)2 Te as precursors [138]. In this ALD process, (Me3 Si)2 Te was initially physically adsorbed on the graphene. The successful low deposition temperature of 70 °C for ~24.0-nm-thick crystalline Sb2 Te3 layer was finally achieved with the crystalline plane parallel to the graphene substrates. A sharp hetero-interface was formed between the graphene and Sb2 Te3 film as depicted on the high resolution transmission electron microscope (HRTEM) image in Fig. 7a [138].
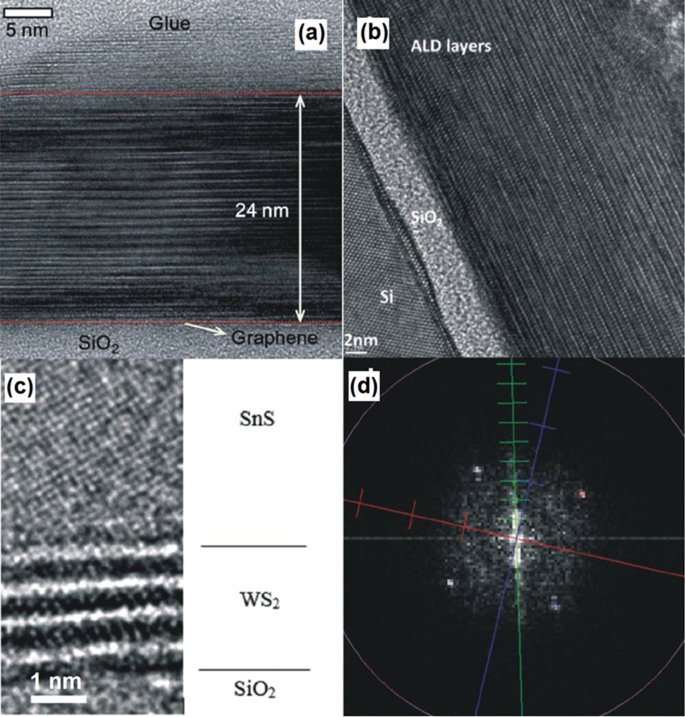
a Cross-sectional image from the interface between ALD grown Sb2 Te3 on graphene/SiO2 Substrat. b The cross-sectional image of heterostructured Sb2 Te3 /Bi2 Te3 stacked layers. c The HRTEM image of SnS/WS2 heterointerfaces and d the corresponding fast Fourier transform (FFT) image. Reproduced with permission from [138]
In another attempt, 2D hetero-structured Sb2 Te3 /Bi2 Te3 metal chalcogenides films were deposited by the ALD process on Si/SiO2 wafer. Here, the bismuth telluride was selected to be deposited over the silicon wafer by the ALD technique at 165–170 °C, since it covers the Si better than Sb2 Te3 . Considering the low ALD temperature of Bi2 Te3 (at 65–70 °C), the employment of initial long SbCl3 pulse in the ALD chamber was taken as a capable strategy to saturate the surface of Bi2 Te3 and complete the surface reactions for the next deposition step [139]. This approach also facilitated the disposition of three stacking layers of Bi2 Te3 /Sb2 Te3 with a high level of crystallinity. Another example is the growth of multiple WS2 /SnS layered semiconductor hetero-junctions on the Si/SiO2 substrates by alternating ALD of WS2 and SnS at the ALD temperature of 390 °C [140]. The stacked 2D WS2 /SnS has the 15° orientation angle between their c axis originated from the difference in crystalline structures and the lattice mismatch between WS2 and SnS (Fig. 7b, c) [133]. This misaligned crystalline characteristics of WS2 /SnS hetero-interfaces is the origin of noticeable decreased of the holes mobility in SnS film [140]. Generally, the ALD techniques are recently undergoing consistent progress to enable the deposition of higher numbers of 2D TMDC films. However, there are still countless research opportunities to develop suitable ALD recipes for deposition of 2D TMDC films over the different substrates and also to develop nanoelectronic instruments based on 2D TMDCs.
ALD of Ultrathin Oxide Films
The few-nanometer-thick metal oxide films can be considered as 2D nanostructures when they can be assigned to few layers of 2D films. In this context, the characterization and exploration of 2D oxides properties for the practical applications are considered as valuable technical knowledge along with the progressive advancement in the device miniaturization. Furthermore, modern technologies use the advantages of ultra-thin metal oxide nanostructures as the main components of state-of-the-art nanodevices. Consequently, promising applications for the ultra-thin 2D semiconductors and insulators are expected, such as solid oxide fuel cells, catalyst films, corrosion protection layers, chemical sensors, spintronic devices, and UV and visible light sensors. Metal oxide semiconductor field-effect transistors (MOSFET) and the other novel nanoelectronic instruments are fundamentally dependent on the ultra-thin uniform films of metal oxides. Complementary, metal oxide semiconductor (CMOS) sensors are technically developed based on the deposition of ultra-thin metal oxide films which opened up a quite number of the different applications from image and light sensors to FET transistors in low-energy semiconductor instruments. The solar energy cells, plasmonic devices, data storage applications, bio-compatibility and bio-sensing features, supercapacitance properties, and electrochemical sensing are among the recently announced and discovered applications of the 2D oxide semiconductors. Thus ALD deposition of 2D metal oxide semiconductor thin films is considered as advanced fabrication technology. It is also an extremely difficult target to achieve considering the fact that the structural stability and molecular integrity of 2D films can be deteriorated easily. Accordingly, one of the main challenges is the versatile and conformal deposition of these 2D oxide nanostructures on the convenient substrates [94]. The following section is specifically focused on the ALD of metal oxide materials, and particular attention is given to molybdenum, titanium, aluminum, and tungsten oxide 2D films.
MoO3
Distinguishable properties of nanostructured molybdenum trioxide (MoO3 ) made it an excellent candidate for catalytic, electrical and optical applications. There are also several promising functionalities originated from the heterointerface between the MoO3 semiconductor and their metallic substrates. The bis(tert-butylimido) bis(dimethylamido) molybdenum ((tBuN)2 (NMe2 )2 Mo) is one of the precursors for MoO3 deposition, since it provides good volatility and thermal stability [141]. The PEALD window for molybdenum oxide with O2 plasma and ((tBuN)2 (NMe2 )2 Mo) precursor is in the range of 50–350 °C. The as-deposited films fabricated at the temperatures below 250 °C are usually amorphous, thus the post-deposition annealing process is needed to experience a transition from the amorphous to crystalline state. While the as-deposited films are sub-stoichiometric, the stoichiometry can be adjusted by the modulation of plasma parameters. The other precursors for ALD of ultra-thin MoO3 film is Mo(CO)6 which is mostly employed via O2 plasma to facilitate the low-temperature PE-ALD of MoO3 films in the narrow ALD window of 152–172 °C [141]. The resulted MoO3 film was deposited with growth rate of 0.75 Å/cycle. The as-deposited film was found amorphous with the oxygen deficiency at the heterointerface between substrate and MoO3 Film. To achieve the crystalline structure, the following post-annealing at temperature above 500–600 °C is required. In another strategy, the ozone plasma was used on the surface of ALD MoO3 film deposited on 300 mm Si/SiO2 wafer [141]. Mo film was fabricated by using Si2 H6 and MoF6 precursors at 200 °C. The wafer-scale deposition of ultra-thin MoO3 film over 4-inch Au on the Si/SiO2 wafer was reported, where C12 H30 N4 Mo as molybdenum precursor and O2 plasma as oxygen source were used at two ALD temperatures of 150 and 250 °C, respectively. The process includes the pre-exposure of bare Au surface by O2 plasma which assists the formation of OH bonding on the Au surface. The following chemisorption of C12 H30 N4 Mo molecules by the active sites on the Au and interaction with OH group on the surface of Au film led to the ligand exchange. The following O2 plasma completed the oxidation process and finalized the growth of ultra-thin monolayer MoO3 film (Fig. 8). The development of Mo–O-Mo bonding is expected after completion of oxidation process. Finally, 4.6-nm-thick MoO3 film was deposited over the wafer substrate. In this technique, the growth rate from 0.78 Å/cycle to 1.21 Å/cycle was measured when the ALD temperature increased from 150 to 250 °C [141].
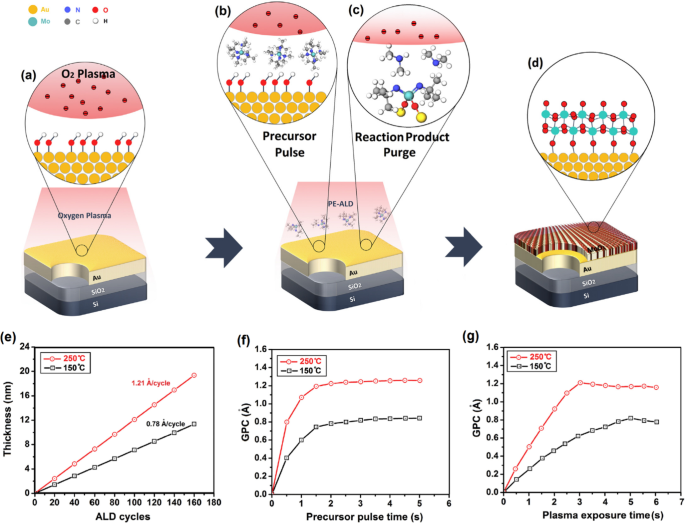
a Graphical scheme of deposition of 2D MoO3 nanofilms by PE-ALD. b The injection of precursor into ALD chamber and c the reaction between precursor and Au surface (d ) and the complete deposition of a monolayer film over Au substrate. e The thickness of PE-ALD MoO3 film over Au substrate vs. the ALD cycle number at based on the usage of (NtBu)2 (NMe2 )2 Mo precursor and O2 plasma at 150 °C and 250 °C, with the precursor dosing time of 2 s and plasma exposure time of 5 s. f The saturation curve of (NtBu)2 (NMe2 )2 Mo precursor. The GPC is demonstrated as the function of precursor dosing time. g The saturation curve for O2 plasma showing the GPC as the function of plasma exposure time. Reproduced with permission from [141]
WO3
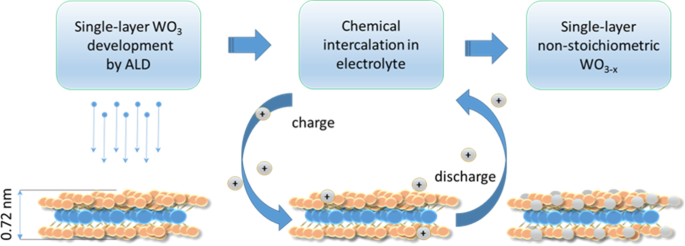
Enhancement of non-stoichiometry in monolayer WO3 film developed by ALD via intercalation/de-intercalation process
TiO2
Wafer-scale deposition of ultra-thin titanium dioxide (TiO2 ) film on the Si/SiO2 substrate was achieved by using the using tetrakis (dimethylamino) titanium (TDMAT) precursor and H2 O as an oxidation agent [145]. The optimum ALD temperature of 250 °C was selected for ALD of monolayer TiO2 film on the wafer-scaled substrate. To achieve a complete coverage of monolayer TiO2 , two individual ALD super-cycles were designed and implemented [145]. The first super-cycle consisted of 10 consecutive cycles of pulse/purge TDMAT to assure the reliable coverage of surface by precursors. Afterward, 10 pulse/purge stages of H2 O were performed to assure the completion of oxidation [145]. The XPS studies confirmed the changes of binding energies in both Si 2p and Ti 2p peaks after the deposition of 2D TiO2 could be elucidated by the development of Si–O–Ti bonds at the interface between native SiO2 and deposited 2D TiO2 Film. The deposition of TiO2 on SiO2 substrate was accompanied by the decrease in binding energy of Si 4+ which was related to this fact that the silicon was more electronegative than titanium. The evidence of oxygen bridge bonding was also demonstrated by characterization of vibrational mode of Ti–O–Ti bonds in the FTIR spectrum of monolayer TiO2 and also by investigation of XPS spectrum of monolayer film [145]. The bandgap of 3.37 eV was measured for this TiO2 film with atomic-scale thickness. The capability of ALD for deposition of ultra-thin films is of great importance to functional applications like microelectronics and catalysis where only few ALD cycles are desirable. Table 3 summarizes practical cases for ALD development of various 2D metal oxides reported to date.
In regard to stability of the ALD fabricated 2D nanostructures and their heterointerfaces, its mainly depends on the surface chemistry of ALD of 2D materials, especially because the inertness of their basal plans is expected to inhibit their growth and thus formation of vdW heterostructures. Moreover, as each precursor has specific “ALD window ” of deposition temperatures, trials and errors approach is required for optimization of the ALD fabrication temperature for each specific precursor taking into account the final thickness and porosity of deposited materials [98, 109]. Owing to the low deposition temperature, ALD of 2D oxides usually leads to fabrication of amorphous layers [32, 94]. Hence, when the crystalline phases of metal oxides are needed, post-annealing treatment is generally applied, improving the crystalline quality of the films as well as the interfaces with the substrate, which ultimately leads to the better stability of 2D nanomaterials over the time. Finally, although ALD appears to be a method of choice to fabricate oxide monolayers due to its precise control of the film thickness, only a handful of reports can be found in the literature [21, 32, 98, 141, 144, 145]. Therefore, the area of 2D metal oxide and their hetero-interfaces fabricated by ALD remains rather unexplored.
Self-limiting 2D Surface Oxides of Liquid Metals
The library of novel 2D materials for advanced applications is currently limited by the conventional synthesis methods of 2D films. The mechanical exfoliation of 2D films from their host layered bulks is not technologically precise and attractive method since the sizes distribution and thickness of produced nanosheets are not adequately and accurately controlled [146]. While the high vacuum techniques are accuracy of the depositing films are considered as high-quality films, these methods are still expensive, time consuming, and restricted by availability of precursors [64]. On the other hand, it is always desirable to introduce new synthesis methods of 2D materials in order to obtain the advanced 2D nanostructures with superior properties. Thus, synthesis of novel 2D semiconductor materials with distinguished or improved characteristics still remains a fundamental challenge.
Room-temperature liquid metals are a group of metals and alloys with unique electron-rich metallic cores which let them to stay in the liquid states even at the room temperatures. The interface of liquid metals with surrounding environment is the origin of one of the most natural 2D films [147]. This self-limiting surface oxide films (skin) is one of the most perfect planar materials with atomic scale thickness [148]. These crystalline structures are the host of unique properties and have provided a novel platform for synthesis of high-quality thin-film 2D materials for advanced applications [149]. Here, the liquid metals have the role of host material for synthesis of 2D films. With respect to the atomic structure, elemental composition, fluidity and thermodynamic of liquid metals, and furthermore, by considering the nucleation and growth characteristics of the surface oxide films, countless parameters are engaged to determine the proprieties of extracted 2D surface oxide films [150]. The low melting monophasic metal alloys are composed of post transition metals (Ga, In, Sn, Pb, Al, and Bi) and the elements of group 12 (Zn, Cd, and Hg). These elements can be combined together to produce a group of liquid alloys with low melting temperature. A biphasic liquid metal (mixture of solid and liquid) can be formed by either deviation of alloy composition from eutectic point or via adding solute elements above the solubility limit into the liquid metal solvent [151]. Gallium (Ga) is one of the most famous liquid metals with room-temperature melting point and possessing both covalent and metallic bonds at the solid state. The strong structural anisotropy and weak atomic bonding between Ga dimmers is originated from the distance between Ga neighbour atoms 2.7–2.9 Å which is considered as a significant distance between the gallium dimers [152]. This weak atomic bonding results in the weak crystalline structure in gallium which can easily break up at the room temperatures [153]. The alloying of Ga with some of the other elements may lead to further decrease in melting temperature of liquid alloy. One of the famous examples is the Ga-In alloy, when the melting temperature decrease to 16 °C in eutectic point of In-Ga alloy with atomic concentration of 14.2% indium [153]. The interface between gallium alloy and surrounding atmosphere is composed of the atomic-scale-thick gallium oxide (Ga2 O3 ). The liquid metal reacts with atmospheric oxygen even at very low oxygen pressure forming a self-limiting metal oxidation reaction [153]. This ultra-planar oxide films are among the most perfect naturally grown 2D materials [61]. This natural film prevents the liquid metal from the further oxidation. The ionic transfer through the natural surface oxide film is the controlling factor for the natural growth of surface oxide films of liquid metal and alloys. The formation of this oxide film leads to the highest reduction in the Gibbs free energy (ΔG f ) [154]. In a liquid metal alloy, the surface oxide film is mostly composed of surface oxide films of one of elemental oxide films of alloy components. The most famous example is the surface of oxide film of galinstan alloy. Galinstan (EGa, In, Sn) is the eutectic alloy of gallium–indium–tin. However, the surface oxide of galinstan is composed of Ga2 O3 . Since the ΔG f of Ga2 O3 is lower than that of In2 O3 und SnO2 in oxygen atmosphere, the surface oxide of galinstan alloy is mostly composed of Ga2 O3 [155]. With the same synthesis strategy, it is possible to synthesize the mixed 2D oxide films similar indium–tin–oxide. To this aim, the molten In–Sn alloy should be used to extract 2D films from the surface of liquid In–Sn alloy [154].
Due to the nonpolar properties of liquid metals, the attraction force between the liquid metal and its natural surface oxide film is weak and localized. Thus, a weak mechanical force can simply delaminate the surface oxide films from the surface of liquid metals. The delamination of surface oxide film can be achieved via the mechanical separation and exfoliation of 2D films by applying mechanical exfoliation methods including sonication, touching of liquid metal with the appropriated selected substrate, mechanical rolling of the liquid metal and alloy over the smooth substrate and separation and extraction of 2D films through the density differences and gradients between the synthesized compounds. These methods were employed recently for delamination of several different types of the surface oxide films of liquid metals from their host alloy. The following section will review some of lately synthesized 2D surface oxide films of liquid metals and alloys.
SnO x
2D SnOx film was successfully delaminated via vdW exfoliation of the liquid tin surface oxide film. Elemental tin (Sn) with its low melting point (231.9 °C) [156] and its high conductivity (9.17 × 10 6 σ) is used as electrical connections in the electronic utensils. The anti-corrosion impact of tin oxide layer has been confirmed before. SnO2 naturally covers the surface of tin alloy under the atmospheric condition and prevents further oxidation of Sn [156]. It was found that the natural surface oxide film of liquid tin in the ambient atmosphere is composed of a mixture of tin oxides which is predominantly composed of SnO2 , with some contributions of SnO, Sn2 O3 and Sn3 O4 [156]. The precise control of oxygen exposure to the surface of fresh molten tin is the main technical factor to determine the composition of surface oxide film of tin alloy. This is a highly important factor during the synthesis of the surface oxide film of molten tin, when we take into consideration the fact that the SnO2 is n- type semiconductor, and SnO is a p -type semiconductor with a variety of different electrical properties. The bipolar characteristics of SnO is another important properties of this material which made it a functional choice for application in electrical invertors [157]. Furthermore, the high stability and sensitivity of well-established SnO films turned them to the desired option for application in chemical-based FET sensors and catalyst films. The transparency is another characteristic of SnO films originated from the wide bandgap of this semiconductor [156, 157].
To exfoliate2D SnOx film, the fresh surface oxide film of molten tin was mechanically delaminated by the contact of surface of Si/SiO2 substrate with the surface of molten alloy inside of the glove box [158]. The scheme of process was graphically demonstrated in Fig. 10a [158]. The delaminated SnOx film in ambient atmosphere had ~ 0.6 nm thickness [157] with crystalline structure. Considering the interlayer spacing of crystalline SnO (0.484 nm), 0.6-nm thick film can be considered as a monolayer SnO film and 1.1-nm-thick film is therefore a bilayer SnO 2D film (Fig. 10b). The 2D films synthesized in glovebox and under oxygen concentration of 10–100 ppm had ultra-fine surface characteristics. The measurement of interlayer spacing showed that the nanosheets formed under ambient atmosphere were composed of two distinct materials with interlayer spacing of 2.7 Å and the other 3.35 Å, which were respectively attributed to the crystal lattice of SnO and SnO2 . The 2.7 Å lattice spacing was related to the (110) plane of SnO, while the 3.35 Å lattice spacing was attributed to the (110) plane of SnO2 , respectively (Fig. 10c–e) [158]. The sample synthesized in the reductive atmospheres was mostly covered by SnO film. The bandgap measurement of SnO film showed the direct bandgap of 4.25 eV. This ultra-thin 2D film was employed to fabricate the SnO-based FET transistor. The p -type characteristics of SnO-based developed FET was confirmed with the mobility of 0.7 cm 2 V −1 s −1 (Fig. 10g) which was much higher than the similar FET devices based on the pulsed layer deposited bilayer SnO film with the mobility of 0.05 cm 2 V −1 s −1 [159].
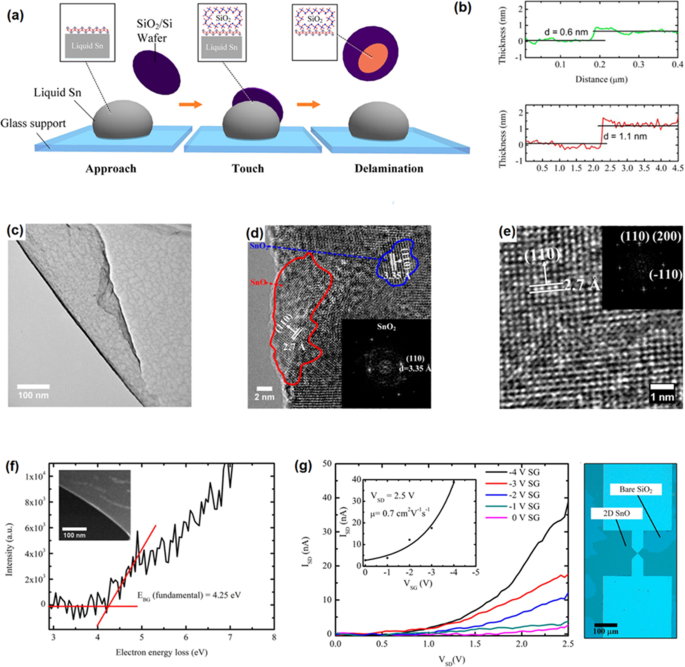
a Graphical representation of vdW exfoliation method. When a Si/SiO2 substrate is attached to the molten tin alloy, the created vdW force between the tin oxide and SiO2 surface help to delamination and following attachment of 2D oxide film to the SiO2 Substrat. b The AFM image of the monolayer (0.6 nm) and bilayer (1.2 nm) tin oxide film. c The TEM image of tin oxide film developed in controlled atmosphere. d The HRTEM of tin oxide film developed in ambient atmosphere with mixed SnO and SnO2 Struktur. e The HRTEM image of SnO film developed in controlled atmosphere. f The bandgap of monolayer SnO film measured by electron energy loss spectroscopy. g The I-V characteristics of fabricated SnO FET device at various gate voltages. Reprinted with permission from [156]
GaPO 4
The high-temperature stable gallium phosphate (GaPO4 ) semiconductor (up to 930 °C) is one of the functional piezoelectric semiconductors with outstanding technical position compared its rival of α-quartz [61]. Gallium phosphate has trigonal structure with the cell parameters of a = 4.87 Å and c = 11.05 Å and γ = 120° (Fig. 11a) [160]. The piezoelectricity facilitates the mutual conversion of electrical energy or pulses to mechanical forces or oscillations. To develop miniature power and electric instruments and generators, it is highly required to synthesize 2D piezoelectric materials for novel nanoelectronic technology. In this device, the atomistic level vibration, bending and displacement can be monitored and then facilitate the harvesting of kinetic energies of oscillation of piezoelectric materials. The loss of centro-symmetry is the main structural specifications of 2D materials with piezoelectric properties [160]. Many types of 2D materials including, TMDCs with odd number of layers, transition metal oxides, aluminium nitride, GeS and SnSe2 , h -BN and graphene are capable of showing the piezoelectric properties [161,162,163] at the low temperatures. Nowadays, it is highly required to synthesize new 2D materials with stable piezoelectric performance at high operational temperatures. This is very challenging issue since the performances of 2D piezoelectric materials are failed due to the structural changes at the high working temperatures. Another difficulty arises from the challenging deposition stage of homogeneous 2D piezoelectric films of functional instruments.
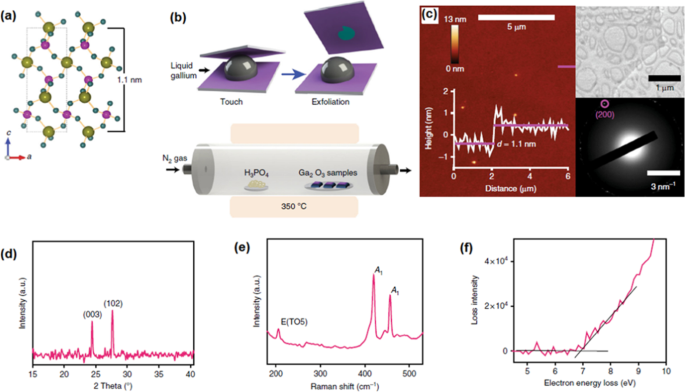
a The side view of GaPO4 demonstrating the out-of-plane structure of GaPO4 . b For the secondary phosphatization treatment. c The AFM and HRTEM studies of 2D GaPO4 Film. d The XRD, e Raman and f bandgap measurement of 2D GaPO4 Film. Reprinted with permission from [160]
As a successful achievement, the 2D archetypal piezoelectric GaPO4 film was recently synthesized via processing of 2D surface oxide film of gallium. The archetypal piezoelectric GaPO4 is not naturally available in crystalline scarified structure [160], and thus the conventional mechanical exfoliation techniques are not capable of synthesis of 2D crystalline gallium phosphate films. In this method, natural surface oxide film of gallium was mechanically exfoliated from its liquid alloy via vdW delamination technique, see Fig. 11b. The secondary post-annealing treatment was employed inside a furnace for the secondary phosphatiation treatment [61]. The vapor gas containing phosphor chemically interacted with the surface of 2D Ga2 O3 film by the heating of H3 PO4 powders. Die N2 gas had the role of carrier gas for transfer of vapor phosphor gas to the surface of 2D Ga2 O3 film at 300–350 °C (Fig. 11b). The following AFM and HRTEM measurement of samples confirmed the synthesis of 1.1-nm-thick 2D film with dimensional characteristics similar to the trigonal GaPO4 in the c crystalline direction (Fig. 11c) [160]. The XRD studies and Raman characterization have confirmed that 2D GaPO4 film had crystalline structured with dominance of (003) and (102) planes of trigonal α-GaPO4 . (Fig. 11d, e). A wide bandgap of 6.9 eV was measured for α-GaPO4 film (Fig. 11f). The experimental measurements reaffirmed the strong out-of-the-plane piezoelectric properties for the GaPO3 2D nanostructures which was around 8.5 pm V −1 [160]. This considerable piezoelectric characteristics of 2D α-GaPO4 highlighted the capability of this synthesis method to develop novel 2D materials with distinguishable mechano-electric properties.
GaN
The same post-processing approach was employed to synthesize GaN and InN 2D films from the surface oxide film of liquid gallium and indium [160]. The technique is based on squeeze printing of liquid gallium droplet between the Si/SiO2 parallel substrates (Fig. 12a). In contact with atmosphere, Ga liquid was instantaneously oxidized in atmospheric condition. The synthesis process resulted in the formation of 2D Ga2 O3 films with thickness of 1.4 nm [157]. The following ammonolysis reaction at 800 °C was operated in the presence of urea. It was found that lower reaction temperatures did not facilitate the conversion of Ga2 O3 into the wurtzite GaN films (Fig. 12b) [160]. The thickness of the film after ammonolysis process was found to be 1.3 nm which is corresponded to the to three wurtzite GaN unit cells (Fig. 12c). The lattice constant of 5.18 nm was measured for 2D GaN film. The TEM studies also confirmed the crystalline structure of GaN with interlayer spacing of 0.28 along the (001) crystalline plate (Fig. 12d) [160]. The X-ray photoelectron spectroscopy (XPS) affirmed the substantial amount of nitrogen was replaced by the oxygen atoms in GaN structure which is owing to the similar bond length of Ga-O and Ga-N. It was targeted to decrease the level of oxygen in 2D GaN films. However, the successful removal of oxygen from the 2D GaN films was not achieved even during the synthesis process at the high temperatures. A bandgap of E g = 3.5 eV was measured for 2D GaN film (Fig. 12e) compared with that of Ga2 O3 Film. The reason for the considerable decrease in the bandgap was attributed to the upward shift of the valence band of GaN film due to the hybridization of the O2p -N2p orbitals [160].
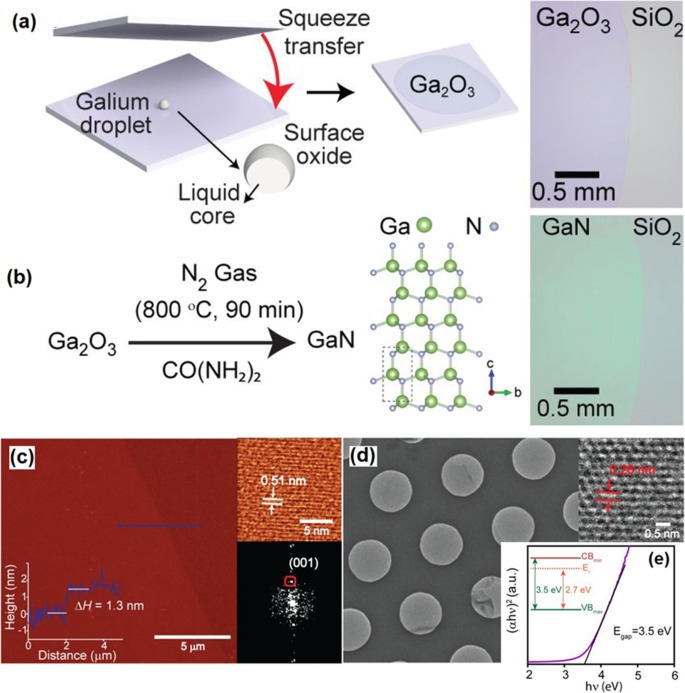
a Schematic illustration of squeezing of liquid metal galinstan between SiO2 substrate and b following post-ammonolysis treatment for phase transform of Ga2 O3 to GaN film. c The High resolution AFM image of the surface of GaN film. d The HRTEM image of GaN film and e the band gap of measurement of GaN film. Reprinted with permission from [160]
The similar strategy was used to synthesis 2D InN film via two-step synthesis technique [160]. In this method, the vdW exfoliated 2D In2 O3 film was extracted from the surface of liquid indium. An intermediate bromination step was performed to transfer In2 O3 to InBr3 . A 20-s post-processing stage of In2 O3 film adjacent to HBr source was enough to complete the phase transformation from In2 O3 into InBr3 [61]. The following ammonolysis treatment of InBr3 2D film by NH3 source at the temperature of 630 °C resulted in the phase transformation of InBr3 into InN [160]. The following characterization studies confirmed the development of crystalline 2D ultra-thin wurtzite InN nanostructures with the crystalline lattice constant of 5.5 Å with the bandgap of 3.5 eV [160]. Generally, the multi-step synthesis technique was found as reliable approach towards the synthesis of advanced 2D nanostructures by using the surface oxide of liquid metals. The developed 2D nanostructures have clearly demonstrated novel electronic and optoelectronic characteristics which are not expected and observed in the similar 2D nanostructures, synthesized via high-vacuum deposition-based methods [164].
SnO/In 2 O 3 2D Heterostructures
The p-n heterojunctions are now the building blocks of the semiconductor devices where the hetero-interfaces between two semiconductor materials with the different types of charge mobility properties are used to fabricate various types of electronic and opto-electronic instruments. Specifically, the heterostructured devices based on 2D films can be developed by several different approaches and techniques including mechanical exfoliation from the host material, CVD, ALD, molecular beam epitaxy, and pulsed laser deposition [165, 166]. Interesting new strategy was recently employed to fabricate vdW heterostructured 2D layered materials via stacking of various 2D layered crystals extracted from their liquid-based metals and alloys. A p-n heterojunction was developed by the vdW exfoliation of 2D SnO and In2 O3 films [167]. In this method, 2D SnO/In2 O3 vdW heterostructures developed [167] by the vdW transfer of surface oxide film of liquid tin (p -SnO) on top of the natural surface oxide of indium (n -In2 O3 ). The developed 2D heterostructured film finally composed of p -SnO/n -In2 O3 2D films attached on top of each other via vdW forces (Fig. 13a, b) [167]. The following microstructural studies in atomic scale levels confirmed the formation of a vdW heterointerfaces between SnO/In2 O3 films with two crystalline structures with the different characteristics fabricated over Si/SiO2 substrate (Fig. 13c). The lattice spacing of 0.27 nm corresponded to the (321) plane of crystalline In2 O3 , and the other 2D film with 0.298 Å is attributed to the (101) plane of SnO. The heterostructured film had the thickness of 4.5 nm (Fig. 13d, e). The Raman and optical characterization studies confirmed the development of heterointerfaces with optical absorption peak of SnO/In2 O3 film located at 251 nm, which was a number between 246 nm (SnO absorption peak) and 256 nm (In2 O3 absorption peak in Fig. 13f, g) [167]. One of the main properties of heterostructured film was strong interlayer coupling of charge carriers which affected the optical absorption of 2D film. The bandgap of heterostructured film was narrowed to 2.30 eV while the bandgap of individual SnO and In2 O3 were 4.8 eV and 3.65 eV, respectively, [167]. The schematic of band alignment at 2D SnO2 /In2 O3 vdW hetero-interfaces was demonstrated at Fig. 13h. The effective bandgap at p–n junction was expected to be 2.30 eV, which was close to the calculated value of band gap of 2D heterostructured film from Tauc plot. The narrow bandgap assisted the charge separation at hetero-interfaces, thus the photoexcited electron–holes can be separated easily and they can feasibly migrate from the valence band of SnO to the conduction band of In2 O3 Film. The specific energy band alignment at the hetero-interfaces of vdW SnO2 /In2 O3 demonstrated quite impressive capabilities and excellent photodetectivity of 5 × 10 9 J with the photocurrent ratio of 24 and the outstanding photoresponsivity of 1047 A/W [167]. Here, the liquid metal printing technique was found as the valuable potential approach for fabrication of heterostructured 2D materials, which do not naturally exist as the layered structures in nature.
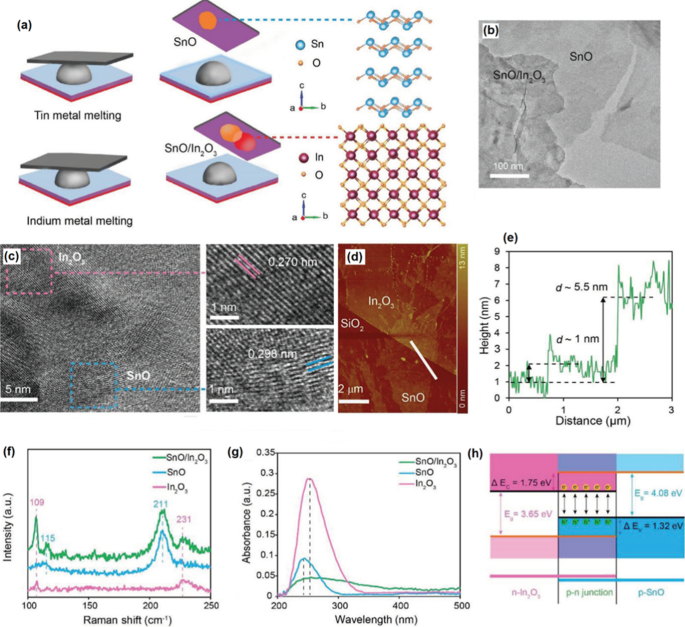
a Schematic illustration of fabrication of 2D vdW heterostructured SnO/In2 O3 Film. b The HRTEM image of heterostructured SnO/In2 O3 Filme. c The HRTEM image of atomic structure of heterostructured films show the high crystalline structure of SnO and In2 O3 film with their lattice spacing distance. d The AFM of heterostructured SnO/In2 O3 film with e the profile thickness of the films. f The Raman spectra of SnO, In2 O3 and SnO/ In2 O3 2D films. g The optical absorption of SnO, In2 O3 and SnO/ In2 O3 2D films. h The band alignment of SnO/ In2 O3 2D heterostructures. Reprinted with permission from [167]
Therefore, the natural ultra-thin metal oxide films on the surface of liquid metals and alloys were found as functional materials with tremendous applications. Ultra-thin nature, pin-hole free and considerable lateral dimensions of these films accompanied by the facile vdW delamination of them on desired substrate provided a great platform for successful synthesize of novel generation of 2D materials and related electronic instruments [168]. Table 4 has provided a representative information on the recently developed 2D materials and devices based on 2D surface oxide film of the liquid metal and alloys.
Novel Applications of 2D Semiconductors
Memristors Based on 2D Semiconductors
The information technology today faces the fast growing of amount of generated data. The data processing in new era requires development of advanced data storage technologies far beyond of concept of current silicon-based technology. Today Si-based memories are facing their technological limits, furthermore, the complementary CMOS instruments are also suffering from the physical dimensional retractions and technological problems [169]. Consequently, it is highly required to develop new nanostructured materials with improved properties and also to modify the existing devices to circumvent the technological restrictions related to the storage capacity of memory units. The von Neumann technology tries to emulate the working mechanism of human brain where the complicated networks of interconnected biological synapse enable us to memorize, learn, remind, recognize, process and understand the multi-task operations with minimum energy consumption. An average power consumption for a singular synaptic event is in the range of 1–10 fj, which is at least 5 orders of magnitude lower than the energy consumption of the existing conventional computers [170]. The current von Neumann technology architecture is not efficient since their memory and processing units are physically separated. A low-energy consumption non-volatile memristors with nanoscale dimensions, high performance, and improved processing speed can respond the current memory technology which is in its bottle-neck stage [170]. Regarding the energy consumption issues, 2D materials can be employed as ultra-thin nanostructural memristors. The resistive switching (RS) behavior of 2D materials has also been explored recently. It was found that the employment of 2D materials in memory units could potentially tackle the current limitations in memory devices [171]. Recently, the nonvolatile characteristics of 2D materials have been extensively investigated and characterized. The 2D semiconductor layered materials and ultra-thin oxide films possess a wide range of properties in various fields which also can be accompanied by their memristive characteristics.
Memristors Based on TMDCs
The recent development in nanodevice fabrication technology enabled the employment of ultra-thin TMDC films in functional instruments. The CVD and metal organic CVD were successfully employed to deposit monolayer MoS2 , MoSe2 , WS2 , and WSe2 2D films in a sandwiched in a vertical configuration between Au electrodes over Si/SiO2 substrate (Fig. 14) [172]. It was confirmed that monolayer TMDC films had no grain boundaries and they are empty of oxide compounds. Thus, an oxide free hetero-interface was shaped between Au electrodes and 2D TMDC films. This sharp and clean hetero-interface increased the possibility of precise measurement of electrical properties of 2D films. The graphical scheme of the memristor devices, the top view of device and the cross-sectional TEM image of Au/MoS2 /Au memristor are presented in Fig. 14a–c, respectively. The characterization of 2D MoS2 by atomic resolution scanning tunnelling microscope (STM) (Fig. 14d) demonstrated relatively high quality and additionally showed local sulfur vacancy defects in the MoS2 Film. The unit based on monolayer MoS2 film verified high on /off ratio above 10 4 and the corresponding retention time as high as 10 6 s. The bipolar resistive switching was observed in the behavior of all memristors based on MOS2 , MoSe2 , WS2 , and WSe2 2D films. Both unipolar and bipolar resistive switching behavior were detected during the study of resistive switching response of MoS2 Filme. The abrupt increase of the device current in all samples indicated the occurrence of dielectric break down phenomenon. The formation of conductive filaments (CFs) in the 2D active layer of memristors was the main mechanism of RS (Fig. 14e–i). Thus, the electroforming can be avoided by scaling down the thickness of active memristor layer into the few nanometer range. The main drawback of this strategy is the excessive leakage current originated from the trap-assisted device tunnelling [165]. Occasionally, the unipolar RS was observed in the certain single-layer MoS2 metal/insulator/metal units as was shown in Fig. 14 f. The typical bipolar RS behavior was also presented in the study of resistive behavior of atomristors based on monolayer MoSe2 , WS2 and WSe2 films, respectively [172].
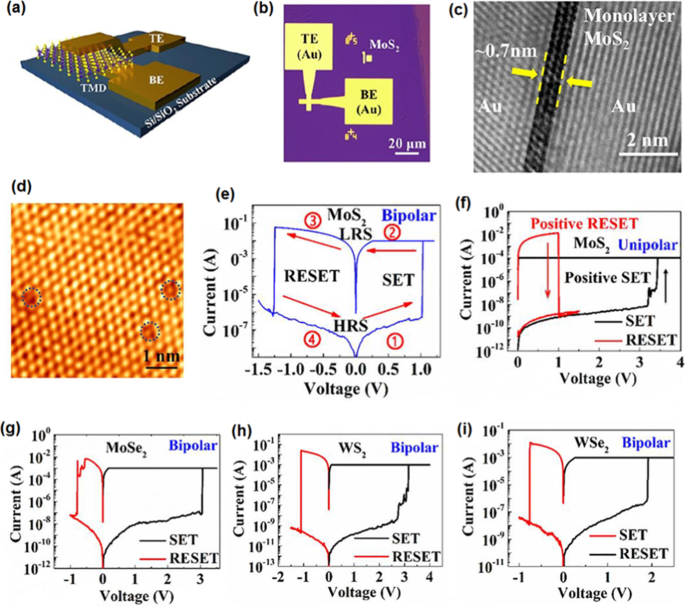
a Schematic interpretation of metal/insulator/metal atomristor device based on TMDCs monolayers, b the corresponding optical image of device and the c cross-sectional TEM image of Au-Monolayer MoS2 -Au device. d The atomically resolved STM image of monolayer MoS2 film deposited on Au electrode. The sulfur vacancies indicated by dashed lines. e The typical I-V curve of atomristor device based on monolayer MoS2 monolayer device. The typical bipolar RS behavior is observed. f The occasional unipolar RE behavior of device in monolayer MoS2 device. g , h and i graphs respectively demonstrate the typical bipolar RS behavior of atomristor devices based on monolayer MoSe2 , WS2 and WSe2 Filme. Reprinted with permission from [172]
In another configuration, a memristor based on monolayer MoS2 was developed where a Cu electrode was employed as the active electrode to fabricate electrochemical metallization memory device [173]. In this instrument, the Au film play the role of inert conductive electrode. The scheme of this device is demonstrated in Fig. 15a. Due to the diffusion of Cu ions in MoS2 layer a conductive atomic-scale Cu filament is formed in the MoS2 double monolayer. Therefore, this memristor falls under the category of electrochemical metallization memories (ECM), whereas the memristive performance of other 2D MoS2 device with the inert electrode (similar Au) is based on migration of the sulfur vacancies. The sulfur vacancies in MoS2 thin films make conductive filaments with atomic scale dimensions, and hence these types of MoS2 memristors are known as the valence change memories (VCM) [173].
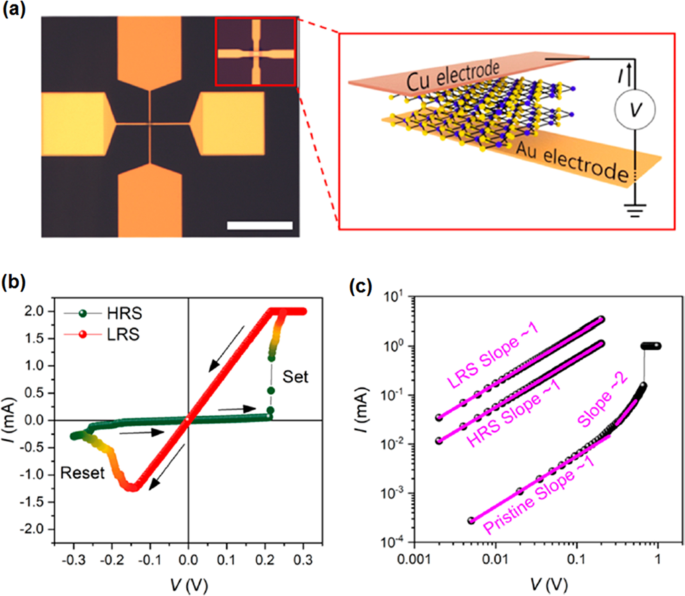
The optical image of Cu/MoS2 double layer/Au memristor device with scale bar of 100 μm accompanied by the graphical scheme of device with top Cu electrode and bottom Au electrode. The memristor’s crossbar area is 2 × 2 μm 2 . b The I-V curve of memristor device with bipolar RS with the 0.25 V Set voltage and − 0.15 V Reset voltage. e The logarithmic scale I –V curve of device for the pristine state before and after forming process, and for LRS and HRS after the forming process. Reprinted with permission from [173]
In fabricated ECM (Fig. 15), the two-dimensionality and electrochemical metallization by Cu cationic filaments resulted in the considerable decrease in switching voltage down to 0.2 V (Fig. 15b). This characteristic has arisen from the lower diffusion activation energy of Cu cations in MoS2 layer compared with that of sulfur vacancies in 2D MoS2 film [173]. The bipolar resistive switching was the main characteristics of the I-V curve of memristor with a set voltage at 0.25 V and a reset voltage at − 0.15 V. It was observed that the majority of double-layer MoS2 memristors are free of the forming process. By using a double-layer MoS2 memristor the transport mechanism in Cu/double layer MoS2 /Ag device was investigated. Figure 15b depicts the I–V characteristics of memristor before the forming process (pristine state) as well as for HRS and LRS (after forming). The instrument performed in pristine state with the resistance of 16.6 kΩ when the applied voltage on the unit was less than 0.3 V. Quantum tunnelling was recognized as the conduction mechanism in memristor in the pristine state. In this condition, the resistance is much larger than the resistance of device after the forming process of memristor. The further increase of applied voltage beyond the 0.3 V in pristine state is accompanied by the transition in I–V curve into the slope 2 region (I ∝ V 2 ), which corresponds to the space-limited conduction states. The dramatic increase of current after increasing of applied voltage (further than 0.6 V) is attributed to the formation of Cu conductive filaments in MoS2 film (Fig. 15c). Higher imposed voltage on Cu electrode resulted in the oxidation of Cu top electrode. This resulted in the migration of Cu cations toward the bottom Au electrode to reduce and deposit there. The conductive Cu filaments were built up along the defective grain boundaries of 2D MoS2 film and the device switched to LRS.
Complementary Resistive Switching in Transition Metal Oxide Films
The ion doping of semiconductor films can tangibly alter the charge transfer mechanism in these ultra-thin films. The memristive characteristics of 2 semiconductors are also tangibly affected by the presence of other foreign ionic species inside active materials of memristors. TiO2 is one of the well-known recognized semiconductors with resistive switching characteristics. A 7.0-nm-thick TiO2 ALD film was used to investigate the impact of ion intercalation in resistive switching of this ultra-thin film [174]. To understand the effect of Indium (In) intercalation in active layer of memristor (TiO2 ), an ion incorporation set up was made. The ion intercalation in this set-up was facilitated by imposed driving voltage on the ion-containing solution covering the surface of 2D TiO2 Film. The potentiodynamic measurements (I–V sweep) of Pt/TiO2 /Au and Pt/In-doped TiO2 /Au memristor under the dark condition showed different RS mechanisms for In-ion doped and non-doped TiO2 Filme. Pt/TiO2 /Au device shows bipolar switching behavior (Fig. 16a). Das V Setzen = 0.94 and V Reset = − 0.98 during I–V sweeping measurement were observed [174]. A bipolar RS behavior of film was explained by the ionic drift of oxygen vacancies owning to employed voltage on the electrochemically inert Au and Pt electrodes. This unit is categorized as VCM cells. The schematic representation of oxygen vacancies distribution for both off and on states are shown in Fig. 16b and c. The dielectric member of memristor is characterized with two parts:low conductive TiO2-x with the thickness of t and insulating part with the thickness of D-t . The applied voltage on the electrode caused the ionic drift of oxygen vacancies, which is accompanied by the shift of border between low conductivity component and insulating part of active layer of memristor. In this condition, the I–V curve of device is not further Ohmic and will be non-linear. In this proposed model, the thickness of insulating layer should be small enough (few nanometer) to let the electric field develops and promotes the ionic drift of defects [174].
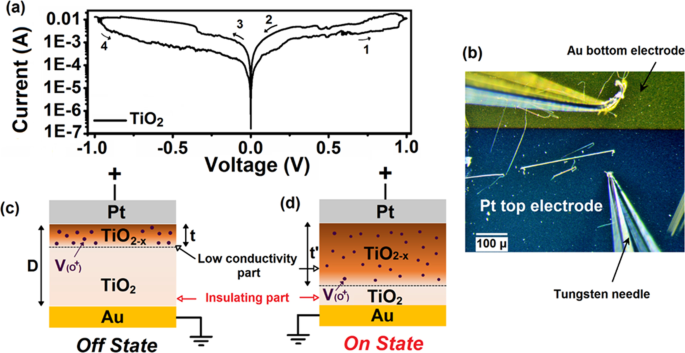
a Typical logarithmic scale depiction of I–V sweeping graph of Pt/In-doped TiO2 /Au memristor. b Device is set off, the metallic cations are distributed in top layer of In-doped TiO2 Film. c The formation of filamentary conductive channels, the device is set On (Set, stage 1). d The depletion of metallic cations adjacent to the bottom electrode. The device is set off again (Reset, stage 2). e By imposing the reverse gate voltage, the metallic cations again move toward the cathode electrode (top Pt electrode) and again form a metallic cation bridge and the device is set On (Set-stage 3). f By depletion of metallic cations, at Pt electrode the device is set off again (Reset, stage 4). Reprinted with permission form [174]
The In-doped TiO2 film demonstrated a complementary resistive switching (CRS) behavior during the voltage sweeping between − 1.0 V and + 1.0 V (Fig. 17a) device is set at a higher voltage and again reset at another lower voltage of the same polarity [174]. The cell was set for the first time at V Set1 = 0.732 V and then reset again in the same polarity at V Reset1 = 0.1277 V. In the negative voltage, the second LRS to HRS switching occurred at V Set2 = − 0.7055 V and again reset to HRS at V Reset2 = − 0.0901 V. Thermally assisted charge transfer mechanism is the most possible theory which explain the resistive switching in the ion-doped TiO2 2D film. The unipolar switching confirms the filamentary nature of resistive switching. The filamentary resistive switching is mostly based on the ECM of the mobile ionic species caused by the migration of metallic cations. It facilitates the development of filamentary metal bridges (on state), which can be ruptured later (off state). The filamentary mechanism is the characteristic of this memristor, which is fabricated with electrochemical active electrodes, while the In-doped TiO2 memristor was sandwiched between inert Au and Pt electrodes. It is expected that the switching behavior of In-doped TiO2 device was originated from the migration of both anion and cation components. The versatile switching behavior of In-doped TiO2 device can prove that both anion and cation components are involved in CRS mechanisms. The proposed model suggested that the redistribution of oxygen vacancies and Indium ions inside TiO2 layer can affect, alter and facilitate the RS. In the heterostructured oxide stacks, the conjunction of an oxygen deficient oxide layer (LRS mode) with another oxygen rich layer (HRS mode) triggers the CRS of heterostructured memristor which is caused by the movement of oxygen vacancies. This gradual change of oxygen concentration can trigger occurrence of CRS of memristor due to the difference in resistance of TiO2 stack. From another point of view the formation of filamentary conductive channels assist the RS and increase the conductance of devise. At the initial state or off state (Fig. 17b), the top In-doped layer is in LRS mode while the bottom layer is in HRS. By applying the threshold voltage, the ionic species migrate into lower insulating layer resulting in the formation of conducting filaments and paths (Fig. 17c) and then device reaches to its on state (Set 1). At higher positive voltage, the ionic species in upper layer (mostly In-ions) are depleted (Fig. 17d) and again device will reach to its off state (Reset 2) and demonstrates HRS behavior. The similar phenomenon is expected to occur at the negative biases in which the first on state (Fig. 17e) switches the memristor to new LRS, following another off state after depletion of the ionic species (Fig. 17f). The versatility of CRS behavior of all samples was repeatedly tested several times and the same behavior was observed.
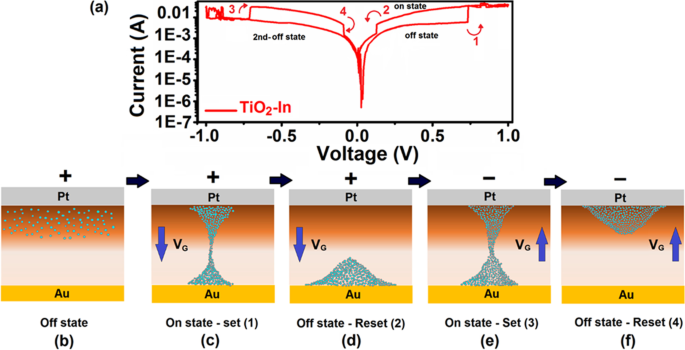
The Pt/TiO2 /Ti/Pt stacked crossbar cells:a the SEM top view of (60 nm) 2 cross-point, b The TEM cross-sectional image of 100 nm 2 Pt/TiO2 /Ti/Pt device with c its corresponding schematic graph of the plug and disc region in the TiO2 Schicht. Reprinted with permission from [174]
Artificial Optical Synapses Based on 2D Semiconductors
The human brain operation is highly energy efficient. The data storage and processing are functioned in brain with the lowest possible energy consumption. This ultra-low energy consumption is inspired by the functionalities of biological nervous system. Based on the brain performance, a novel type of computing and processing system was proposed by Alan Turing in 1948, when he introduced the idea of using a computing device with the interconnected network of artificial neurons [175]. It is envisaged that the interconnect network of artificial synapses can effectively solve and overcome the restrictions of the traditional van Neumann computing. The biological synapses, as one of the key members of the neural system, are the information channels ensuring short-term computation, long-term learning and memorization by tuning the synaptic weights [176]. As a biological structure, a chemical synapse is a gapped connection between two neurons through which the communication between two individual axons is created by biochemical reactions. The synapse is a biological junction where the electrochemical waves (action potentials) are transmitted through the axons of neuron. The energy consumption of a biological synaptic event is tangibly lower than that of any other similar device. Analogously, an artificial synapse is a unit which mimics the behavior and performance of the biological synaptic junction to transfer the synaptic signals. From the technical point of view, memristors are the best candidates capable of successful emulation of the characteristics of a biological synapse [177]. The resistive switching mechanism is the basic principle of the memristors. A sandwiched insulator or transition metal oxides between two conductive electrodes is the typical structure of an artificial synaptic device. The synaptic properties are observed in variety of materials with different resistive and synaptic mechanisms. In fact, the diversity of materials with synaptic characteristics experiences a rapid progress, and thus the fundamental understanding of related synaptic mechanisms is currently progressing. Metal oxide resistive random access memories (RRAMs) (such as TiO2 , AlOx , HfOx , WOx , TaOx ), conductive bridge synapses (Ag- and Cu-based electrodes), phase-change material-based synapses (PC synapse), ferroelectric material-based synapses, magnetoresistive (MR)-based synapses, carbon nanotube based synapses, and organic nanostructured-based synapses are some of the active memristor materials that demonstrated attractive synaptic characteristics [177]. The optical stimulated synaptic devices are recently introduced into telecommunication technology. In optical synapses, optical pulses of light stimulate the generation of charge carriers between two conductive electrodes. The photogenerated charge carriers in atomically thin 2D MoS2 film allow the modulation of the synaptic weights with photonic pulses. This optical capability of artificial synapse is highly important that capitalizes the possibility of high-bandwidth optical communication protocols. The schematic of 2D MoS2 -based synaptic unit is depicted in Fig. 18a [178]. As the main privilege, the incorporation of the optical and electrical pulses addressed the different charge trapping mechanisms to precisely modulate the synaptic weights. Furthermore, the instrument was capable of neuromodulation to achieve the plasticity and metaplasticity properties via dynamic control of spike-time-dependent plasticity (Hebbian theory). The schematic of analogue circuit is demonstrated in Fig. 18b depicting the interplay between Hebbian and homeostatic plasticity. The interplay between Hebbian and homeostatic synaptic plasticity in MoS2 synapse is provided in Fig. 18c–e, respectively. The long-term potentiation strength was achieved by employment of sequential voltaic training pulses in synaptic instrument. It was observed that the synaptic weights increase linearly by increasing the number of training pulses. It was also demonstrated that the application of 100 training pulses resulted in the weight change of synapse up to 117% in electronic mode, while the same number of training pulses in ionotronic-mode resulted in the increase of weight up to 223%. (Fig. 18c, d) [178]. In the optically stimulated synaptic unit, a strong long-term potentiation was observed in the 2D MoS2 artificial synapse via using the optical gating function (Photoactive mode). The light pulses (λ = 445 nm) played the role of presynaptic signals. Up on the illumination on the surface of 2D MoS2 synapse, the conductance increased rapidly and then continued until the gradual saturation when the conductance was one order of magnitude above the value of dark-current. A rapid drop of conductance occurred on the termination of optical illumination source, but the conductance still remained in high values (Fig. 18e). The band-to-band transition is expected to be the main reason for rapid transition, while the long-lasting high conductance values in optoelectronic mode were attributed to the defect or trap-centred slow recombination of generated electron/holes. The observations are in accordance with the proposed model of random local potential fluctuation (RLFP). This state is called persistent conductivity [178]. By the increase of optical pulses and higher number of photons, the decay time became slower. This is consistent with the RLPF model where higher carrier numbers occupy sites of the local potential minima, and therefore, the rate of election/hole recombination would be slower. The vivid manifestation of this phenomenon is slower degradation of photogenerated current in synaptic device.
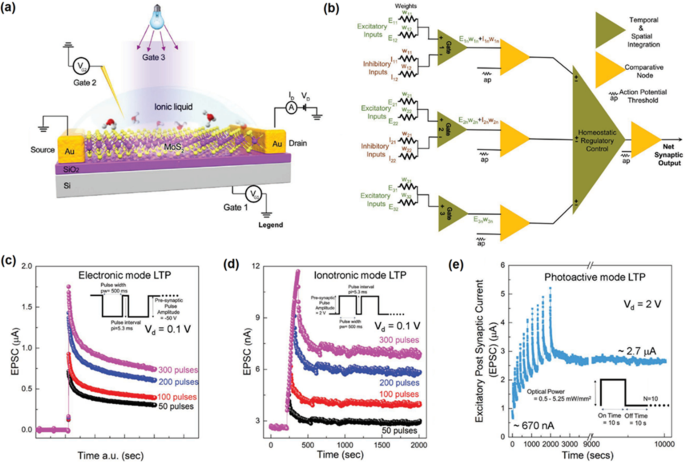
Graphical scheme of triple modulated architecture of artificial 2D MoS2 synaptic device. b The analogue circuit depicting the interplay between the various parameters for stimulation of synaptic plasticity. c The interplay between the Hebbian and synaptic plasticity in MoS2 -based 2D synaptic device, which depicts the enhancement in the strength of long-term potentiation functionalities of device as he function of training by electronic-mode, d Ionotronic mode and e photovoltaic mode. In photovoltaic mode, the light intensity is altered (from 0.5 to 5.25 mW mm −2 ) to enable training by optical pulses. Reprinted and reproduced with permission from [178]
Two-terminal optical synapse based on In-doped TiO2 ultra-thin film was also fabricated for emulation of the synaptic functionalities [174]. The In ions were doped into ~7.0-nm-thick TiO2 film deposited by ALD technique. It was observed that the ion doping affected the RS mechanism and also extended the optical absorption edge of ultra-thin 2D TiO2 film to the visible light range. It was measured that the set voltage of the TiO2 -based synaptic device was shifted to the lower voltages after optical stimulation of In-doped TiO2 memristor. These observations (Fig. 19a) confirmed the impact of photogenerated electron/hole on occupation of trapping sites in amorphous TiO2 film and consequently resulted in the decrease of the set voltage (Fig. 19b) and the increase of memristors’ conductance (Fig. 19b). Furthermore, the increase in light intensity slightly affected the set voltage of memristors, which is an indication of saturation state in the number of photogenerated charge carriers. Therefore, it does not let the device to achieve the lower V Setzen Werte. The emulation of optical synaptic characteristics was verified by the observation of unrested postsynaptic current caused by singular optical pulse (Fig. 19c). PPF synapse values were demonstrated by two consecutive EPSC spikes with pulse intervals of 0.3 s (Fig. 19d). The rapid decay of PPF index of device [the ratio of amplitude of the 2nd EPSC (A2 ) to the 1st EPSC (A1 )] was discovered after the increase of pulse intervals. It shows the sensitivity of short-term plasticity of optical synapses to the sequence of optical pulses (Fig. 19e). The calculated energy consumption of In-doped TiO2 optical synaptic device for a singular pulse was estimated to be around 2.41 pJ (pulsed optical signal with 10 ms duration) (Fig. 19e). It was observed that the energy consumption of In-doped ultra-thin TiO2 optical synapse was highly efficient [174]. Moreover, when the successive laser pulses with different frequencies were employed, transition from STP to LTP emulated by the same optical synaptic unit (Fig. 19f). These results clearly demonstrated that the optical stimuli with the lower pulse intervals are beneficial for facilitation the LTP capabilities. Observations also confirmed that shorter pulse intervals resulted in higher gain values consistent with the effect of residual generated carries on the following pulses [174]. The EPSC and conductance saturation were also detected and observed during the study of synaptic behavior of artificial synapses. This phenomenon was attributed to the saturation of photogenerated electron/holes. It was also shown that the instrument was capable of emulation of bidirectional analogue switching.
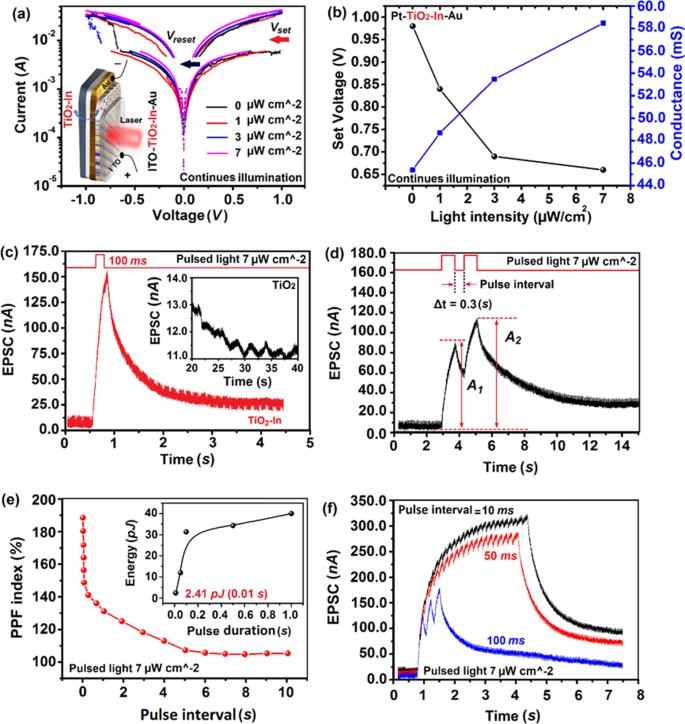
The optical synaptic characteristics of In-doped ultra-thin TiO2 Filme. a The logarithmic scale I–V curves of ITO/In-doped/Au synaptic device. Variation of the set voltage and conductance vs changes of light intensity for pulsed lasers. c The EPSC of synaptic device induced by 7 μW cm −2 laser pulse. d The PPF of device. e The variation of PPF index vs. the pulse intervals. Inset shows the variation of energy consumption vs. pulse duration. f The variation of EPSC of synaptic device stimulated with the pulsed light with different pulse intervals. Reprinted with permission from [174]
Artificial Optical Nociceptors Based on 2D Semiconductors
Mimicking the human brain functionalities by using neuromorphic-based technologies is quite essential achievement towards the development of the artificially engineered bio-inspired electronic instruments [52]. The emulation of human sensory system and the sensorimotor functionalities are significant hurdles in biomimetic studies. Visual processing is fulfilled by outstanding features of the human’s eye (Fig. 20a) [52]. Eye, as the natural visual detector and processor consists of a large number of receptors and nociceptors (Fig. 20b–d). In fact, nociceptor is a key sensory receptor that recognizes noxious stimuli, which in turn generates and delivers the warning signals to the central nervous system. The cornea has the highest number of nociceptors in the human eye and the majority of the corneal nociceptors are polymodal. Looking at the nervous system, the biological synapse is the fundamental base of sensorimotor system facilitating various functionalities including the pain signal transfer in the neural system (Fig. 20e). The analogous artificial nociceptive device with similar synaptic functionalities is composed of a semiconductor ultra-thin film sandwiched between two conductive layers (Fig. 20f).
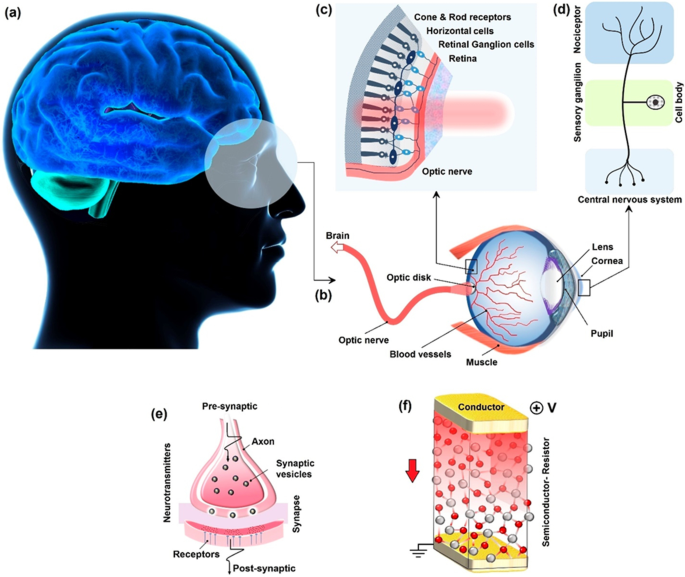
Scheme of human eye receptor and nociceptor system. a The human brain as decision making unit receives the informative signals from b human eye and its sensory components including the c light receptors and nociceptors in Retina section. d Shows a typical nociceptor with its components. e Is the typical schematic representation of a natural synapse and f its artificial counterparts in conductor/ semiconductor /conductor sandwiched configuration. Reprinted with permission from [52]
Optical artificial nociceptors built upon ultra-thin amorphous all-oxide heterostructures with ionic transport, anisotropic electrical characteristics and ultimate transparency. A heterostructured 2D Ga2 O3 /TiO2 film was fabricated to make an optical nociceptor device [179]. To alter the heterointerfaces between Au electrodes and TiO2 optical semiconductor films, the Ga2 O3 2D film was annealed in N2 Atmosphäre. The successful incorporation of N2 atoms into ultra-thin Ga2 O3 film was accompanied by phase transformation of Ga2 O3 into more conductive phase. Raman spectroscopy measurements showed the characteristic peak of Ga-N bonding after annealing process in N2 atmosphere [179]. Der Ga2 O3 film was still in high level of transparency (up to 90%) [179]. From the PL spectra, it is found that the bandgap of 2D WO3 is around 2.88 eV. The PL spectra also showed notable modification of bandgap after RTA of Ga2 O3 2D film in N2 Atmosphäre. The bandgap of 5-nm-thick Ga2 O3 film was shifted from 4.1 eV to 3.46 eV for N2 doped Ga2 O3 Film. It was observed that the oxygen vacancies were replaced by atomic nitrogen in the Ga2 O3 nanostructure. The heterointerface engineering of 2D Ga2 O3 (N2 )/TiO2 altered the resistive switching mechanism of 2D heterostructured memristor film [179]. A self-rectifying behavior was observed during the measurement of optical resistive behavior of heterostructured films. The studies show how the N2 incorporation has considerably altered the energy band alignment at the Ga2 O3 /TiO2 hetero-interfaces [179]. Thus, the charge transfer mechanisms at Ga2 O3 :TiO2 and Ga2 O3 (N2 )/TiO2 hetero-interfaces are expected to be different. A bipolar resistive switching performance was observed during I–V cyclic test of Au/Ga2 O3 –TiO2 /ITO device under the visible light illumination. The tunable HRS/LRS ratio and loop opening characteristics are the main privileges of Au/Ga2 O3 /TiO2 /ITO optical memristor under the visible light illumination. Der Ga2 O3 (N2 -600 °C)/TiO2 heterostructured device has experienced strong rectification behavior which is a characteristic of the development of type-II heterointerface [52, 179, 180]. Thus the main characteristic of this device is its nanoscale ultra-low memristive current, which shows the capability of this unit for the fabrication of the self-rectifying resistive switching-based instruments with low-energy consumption. The nociceptive behavior was observed during the study of reaction of Au–Ga2 O3 /TiO2 –ITO and Au–Ga2 O3 (N2 -600 °C)/TiO2 -ITO. The ignition time (t 0 ) and saturation time (t s ) of the Ga2 O3 (N2 )/TiO2 -based nociceptors are shown in Fig. 21a [179]. The relaxation phenomenon is another characteristic of nociceptors, which was observed in the Ga2 O3 (N2 )/TiO2 -based heterostructures (Fig. 21b). After the nociceptor ignition the ultimate time for the retrieval of nociceptor to off state is called relaxation time. This behavior is similar to the non-adaptation characteristics of the human nociceptors, when the generated warning signals protects the human organs from further unnecessary exposure to hazardous stimuli [180, 181]. It was also found that the relaxation time is the function of the frequency of pulsed light. The abnormal state is a condition when a nociceptor component experiences stimulating signals which are stronger than its natural threshold. In the abnormal state the nociceptor performs similar to the receptor system. Figure 21c shows the difference between the optoresponsive reaction of an abnormal and normal nociceptor. It depicts how the damaged nociceptors show reaction to the constant visible light. The allodynia and hyperalgesia are the individual characteristics of damaged nociceptor in te abnormal state. The response of nociceptor is continuously increased and the device did not follow the non-adaptation mode when the light intensity is increased (Fig. 21d) after 5 min illumination, which is the characteristic behavior of nociceptors in the abnormal state [179]. Generally, it was found that the nanoengineering and functionalization of semiconductor hetero-interfaces facilitated the fabrication of smart bio-inspired photoreceptors and nociceptor gadgets with self-adapting characteristics.
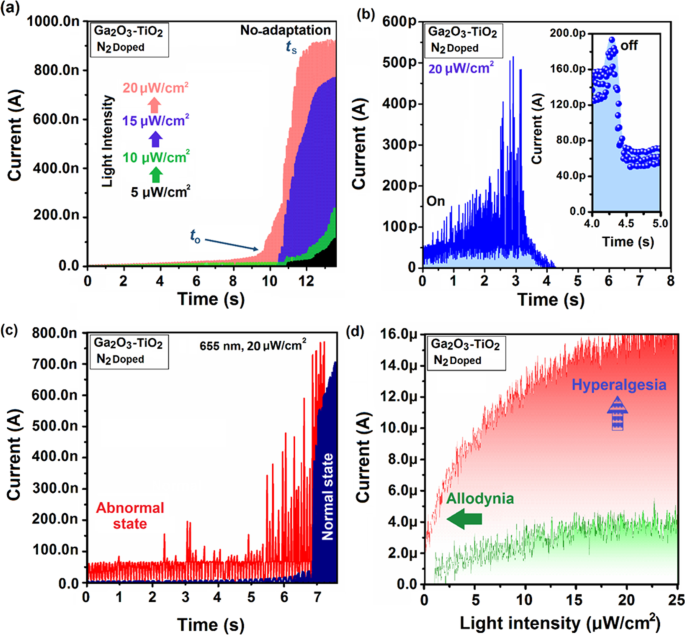
a The photoinduced nociceptive behavior of Ga2 O3 (N2 )/TiO2 -based heterostructured devices. The effect of light intensity (λ = 655 nm) on threshold time (t 0 ) and saturation time (t s ) of Ga2 O3 (N2 )/TiO2 nociceptor. The light frequency was 20 Hz. b The relaxation characteristics of Au- Ga2 O3 (N2 )/TiO2 -ITO nociceptor. The light frequency was 20 Hz. c The distinction of photoresponse behavior of Au-Ga2 O3 (N2 )/TiO2 -ITO nociceptor in normal and abnormal state. The light frequency was 20 Hz. d The allodynia and hyperalgesia behavior of Au-Ga2 O3 (N2 )/TiO2 -ITO opto-nociceptor. Reprinted with permission from [179]
Schlussfolgerungen
The present review has outlined the most recent advancements in the development of wafer-scaled 2D semiconductor nanomaterials for the practical devices fabricated by CVD and ALD techniques. Each of these techniques has its own unique features, merits, complexity, architectures and attractiveness. Despite the rapid progress in the deposition techniques of 2D materials it is fair to say that this field still is in progressing stage. Absolutely new functional opportunities can be initiated and launched if the next generation of advanced functional instruments will be based on 2D semiconductors. Specifically, the outstanding performance of these devices can be achieved through the combinatorial multi-stacking layered 2D nanomaterials. Considering that each of 2D materials has quite different functionalities, multi-functional nanomaterials can be created with enormous advantages in terms of energy and volume. It can be envisaged that a library of 2D materials and nanosheets is required to be updated and renewed regularly. Furthermore, the library of 2D materials is required to be extended by using innovative methods for synthesis of 2D materials from novel material sources.
Moreover, some of the drawbacks of 2D materials fabrication mentioned in review can be solved by obtaining further knowledge about nanointerfaces formation. In this case, the nucleation step and the epitaxial growth has to be controlled in order to avoid or tune eventual interface layer and defects at the interface as well as the mechanical stress and the lattice deformation that can occur in vdW heterostructures. Such stacking imperfections have drastic effects on the physical properties, and therefore, must be addressed for implementation of the final structures. Moreover, the relative rotation angle between both layers directly influences the elastic deformations and hence their tuning permits tailoring the electronic properties of the whole structure. In this regard controlling stack orientation of the ALD fabricated 2D heterostructures would allow the fabrication of 2D materials that have not yet been explored and stipulate new pathways for 2D electronic device fabrication, such as FETs, memristors, resistive switching memory cells and microelectromechanical systems. To enable controlled growth of successive ALD 2D layers, surface functionalization (chemical or physical ) might be required. Despite eventual vdW induced growth, lack of dangling bonds on the 2D surface may inhibit the subsequent deposition. Consequently, the specific functionalization could be realized in order to allow the ALD nucleation while preserving the intrinsic properties of the underlying material.
For further progress in ALD fabrication of various 2D semiconductors, novel ALD precursors must be developed allowing broader expansion of ALD technique in the field of 2D nanomaterials. In this regard, several predicted 2D materials, as silicene, germanene, phosphorene, as well as telluride have not yet been ALD fabricated owing to the lack of suitable precursors. It should also be pointed out that even though graphene is the most studied 2D material, its ALD is still challenging, the only ALD process available leading to material quality inferior to the one obtained using CVD technique.
Finally, one of the main challenges highlighted in many recent published reports is the transition of 2D nanomaterials and their heterostructures into various industrial applications. Currently, only CVD produced graphene is under such transition. Thus, one can envisage that progresses of ALD and CVD of 2D nanomaterials, in particular direct growth of the wafer scale without grain boundaries on integrable devices, will enable their fast industrialization and comercialization.
Verfügbarkeit von Daten und Materialien
Not applicable.
Abkürzungen
- ALD:
-
Atomlagenabscheidung
- Lebenslauf:
-
Chemische Gasphasenabscheidung
- SEM:
-
Rasterelektronenmikroskopie
- EDS:
-
Energiedispersive Spektroskopie
- XPS:
-
Röntgenphotoelektronenspektroskopie
- UV–vis:
-
Ultraviolet–visible
- FTIR:
-
Fourier Transform Infrared
- PL:
-
Photolumineszenz
- TMDCs:
-
Transitional metal dichalcogenides
- vdW:
-
Van der Waals
- COFs:
-
Covalent organic frameworks
- FET:
-
Field-effect transistor
- HRTEM:
-
Hochauflösende Transmissionselektronenmikroskopie
- VCM:
-
Valence change memristor
- ECM:
-
Electrochemical metallization memories
- CRS:
-
Complementary resistive switching
- MR:
-
Magnetoresistive
- RLFP:
-
Random local potential fluctuation
Nanomaterialien
- Eigenschaften und Anwendungen von Tantal
- Eigenschaften und Anwendungen von Titan
- Anwendungen von Molybdän und Molybdänlegierungen
- Elektronen und „Löcher“
- Arduino-Sensor – Typen und Anwendungen
- Funktion und Anwendungen des Fingerabdrucksensors
- Funktion und Anwendung von Schwingungssensoren
- Anwendungen und Einschränkungen des genetischen Algorithmus
- Vorteile und Anwendungen von Rapid Prototyping
- Industriebremsen:Zweck und Anwendungen