Auf dem Weg zu TiO2-Nanofluiden – Teil 1:Vorbereitung und Eigenschaften
Zusammenfassung
Als neue Generation von Arbeitsflüssigkeiten galt Nanofluid in den letzten drei Jahrzehnten schon lange als heißes Forschungsthema. Viele Übersichtsartikel haben umfassende und systematische Zusammenfassungen über die Entwicklung und den Stand der Technik von Nanofluiden geliefert. Eine umfassende Übersicht über alle Arten von Nanofluiden wird heute aufgrund der Fülle an einschlägiger Literatur immer schwieriger. Und viele Kontroversen und Inkonsistenzen in den berichteten Argumenten wurden in verschiedenen Nanoflüssigkeiten beobachtet. Inzwischen sind die systematischen oder umfassenden Übersichten zu einer bestimmten Art von Nanofluid unzureichend. Daher konzentriert sich dieser Bericht auf die Forschung über eine der heißesten Arten, nämlich. TiO2 nanofluid, das aufgrund seiner interessanten und umfassenden Eigenschaften wie sensationelles Dispergiervermögen, chemische Stabilität und Ungiftigkeit große Aufmerksamkeit der Wissenschaftler auf sich gezogen hat. Da die Herstellung von Nanofluiden die Voraussetzung ist und physikalische Eigenschaften kritische Faktoren für weitere Anwendungen sind, fasst dieser erste Teil der Übersicht die neuere Forschung zur Herstellung, Stabilität und physikalischen Eigenschaften von TiO2 . zusammen Nanoflüssigkeiten.
Rezension
Hintergrund
Entwicklung von Nanofluiden
Da die Wärmeübertragungskapazität von Flüssigkeiten im Allgemeinen weit unter der von festen Metallen oder Metallverbindungen liegt, wird erwartet, dass der Wärmetransport von Flüssigkeiten durch das Einschwemmen von Feststoffpartikeln verbessert werden kann. Bei Suspensionen mit Millimeter- oder Mikrometer-Partikeln traten jedoch einige Nachteile auf, wie z. und sogar Rohrblock. Eine neue Möglichkeit, diese Nachteile zu überwinden, wurde gefunden, als eine neue Generation von Aufhängungen, nämlich. Nanofluid wurde 1995 von Choi vorgeschlagen [1].
Nanofluid ist eine neuartige verdünnte Suspension, die Nanopartikel enthält, deren mindestens eindimensionale Größe unter 100 nm liegt. Wenn die Partikelgrößen in der Suspension den Nanometerbereich erreichen, wird erwartet, dass die Suspension eine bessere thermische Eigenschaft erreichen kann und gleichzeitig stabiler bleibt als Millimeter- oder Mikrometer-Partikel/Flüssigkeitsmischung. Ein stabiles Nanofluid kann auch eine bessere Liquidität erhalten und manchmal kann es als einphasiges Fluid behandelt werden. Daher ist eine der größten Herausforderungen für Nanofluide die Herstellung und Stabilität, die die Hauptvoraussetzung für das Erreichen guter thermophysikalischer Eigenschaften und weiterer technischer Anwendungen sind. Dementsprechend lässt sich die Forschung zu den Nanofluiden generell in folgende Richtungen einteilen:Präparations- und Stabilitätsuntersuchung [2, 3], physikalische Eigenschaften wie Wärmeleitfähigkeit [4,5,6,7,8] und Viskositätsanalyse [9,10 ,11,12], Wärmeübertragungsforschung [13, 14], technische Anwendung [15,16,17,18] und theoretische Analyse oder Modellentwicklung [19,20,21,22,23,24,25].
In den letzten zwei Jahrzehnten, insbesondere in den letzten 10 Jahren, hat die Forschung über Nanoflüssigkeiten aufgrund ihrer faszinierenden Eigenschaften explosionsartig zugenommen und viele Forscher haben experimentelle oder theoretische Studien zu verschiedenen Aspekten von Nanoflüssigkeiten durchgeführt [26,27,28,29]. Um dies zu veranschaulichen, ist in Abb. 1 der Wachstumstrend der Zahl der Veröffentlichungen zu sehen, die „Nanofluids oder Nanofluid“ im Titel enthalten, die aus dem „Web of Science“ abgerufen wurden. Diese Abbildung zeigt deutlich, dass die Forschung zu Nanofluiden so schnell wächst, dass die Veröffentlichung im Jahr 2016 hat in den letzten zwei Jahrzehnten 21,9% der Gesamtzahl erreicht. Würde der Suchumfang auf Volltext gelockert und mehr Suchdatenbanken enthalten, könnten sich die Ergebnisse um ein Vielfaches erhöhen. Daher wird es aufgrund der Fülle an einschlägiger Literatur immer schwieriger, einen umfassenden Überblick über alle Arten von Nanofluiden zu geben. Und in den letzten 2 Jahren haben sich einige Rezensionen auf einen Aspekt von Eigentum oder eine bestimmte Art von Nanofluid konzentriert, um umfassendere Überprüfungen zu ermöglichen. Tabelle 1 zeigt beispielsweise die neuesten Rezensionen zu einigen speziellen Aspekten von Nanoflüssigkeiten wie:
- (1)
Vorbereitung oder Charakterisierung [30,31,32]
- (2)
Bestimmte Arten von Nanopartikeln (Al2 O3 , TiO2 , CuO, Graphen, CNT, hybride Nanoflüssigkeiten) [32,33,34,35,36,37,38]
- (3)
Bestimmte Arten von Basisflüssigkeiten (Wasser, EG, EG/Wasser-Gemisch, Öl) [39,40,41,42]
- (4)
Eine oder mehrere physikalische Eigenschaften (Wärmeleitfähigkeit, Viskosität, spezifische Wärme) [43,44,45,46,47]
- (5)
Bestimmte Arten von Eigenschaften (erzwungen, Natur, Siedekonvektionswärmeübertragung, Druckabfall, Partikelmigration) [48,49,50,51,52,53]
- (6)
Einige spezielle Anwendungen (Wärmetauscher, Sonnenkollektoren, Kühlung) [54,55,56,57,58,59,60,61,62]
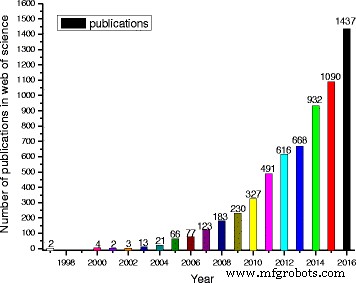
Anzahl der Veröffentlichungen mit „Nanofluids or Nanofluid“ im Titel, abgerufen aus „Web of Science“
Vorteile von TiO2 Nanoflüssigkeiten
Die obigen Einführungen in Tabelle 1 zeigen die Durchführbarkeit und Bedeutung von Übersichten zu einigen speziellen Richtungen von Nanofluiden, da sie relativ umfassende und detaillierte Informationen für einen bestimmten Aspekt liefern können. Als eine der am weitesten verbreiteten Arten ist TiO2 Nanofluide haben aufgrund ihrer hervorragenden physikalischen und chemischen Eigenschaften große Aufmerksamkeit der Wissenschaftler auf sich gezogen. Erstens TiO2 wird häufig in den Bereichen Druck, Kosmetik, Luftreinigung usw. verwendet und ist ein allgemein anerkanntes sicheres Material ohne jegliche Toxizität für den Menschen. Angesichts der Sicherheit dieses Nanofluids haben Taghizadeh-Tabari et al. [63] haben TiO2 . aufgetragen –Wasser-Nanofluid in einem Plattenwärmetauscher für die Milchpasteurisierungsindustrie. Zweitens TiO2 hat außergewöhnliche chemische Stabilität, Beständigkeit gegen Säure-, Alkali- und die meisten organischen Lösungen. Drittens TiO2 Nanopartikel wurden in größerer industrieller Qualität hergestellt, was sie relativ wirtschaftlich macht [64]. Viertens TiO2 Nanopartikel haben eine relativ gute Dispensierbarkeit sowohl in polaren als auch in unpolaren Basisflüssigkeiten, insbesondere wenn ein geeignetes Dispergiermittel zugegeben wird. Yanget al. [65] untersuchten die Dispersionsstabilität von 20 Arten von Nanopartikeln in Ammoniak-Wasser-Lösung. Die Ergebnisse zeigten, dass Anatas TiO2 war das stabilste Metalloxid ohne Tensid, und seine Stabilität konnte durch Zugabe eines geeigneten Tensids weiter verbessert werden. Im Bericht von Silambarasan et al. [66] wird die Absorptionsfähigkeit von TiO2 Nanoflüssigkeiten variierten nach 10 Tagen Lagerung sehr wenig, wie in Abb. 2 gezeigt. Eine solche geringfügige Änderung der Saugfähigkeit weist darauf hin, dass die Stabilität von TiO2 Nanoflüssigkeiten, die sie herstellten, war ziemlich bemerkenswert. Durch die Zusammenfassung der verfügbaren Literatur kann geschlossen werden, dass TiO2 Nanopartikel haben im Allgemeinen eine bessere Dispensierbarkeit als andere herkömmliche Metalloxid-Nanopartikel. Da die Dispergierung von Nanopartikeln in Flüssigkeit die wichtigste Voraussetzung für die Anwendung von Nanofluiden ist, haben sich viele Forscher für TiO2 . entschieden Nanofluide als Forschungsgegenstand.
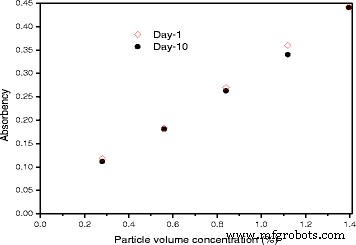
Extinktion als Funktion der Partikelvolumenkonzentration Tag 1 und Tag 10 [66]. Reproduziert mit Genehmigung von Elsevier
Da die Herstellung von Nanofluiden die Voraussetzung ist und physikalische Eigenschaften kritische Faktoren für das Design und den Bau von energiebezogenen Anwendungen sind, ist das Ziel der beiden Übersichtsartikel, die jüngsten Studienfortschritte zu TiO2 . systematisch zusammenzufassen Nanoflüssigkeiten, einschließlich der Herstellung, Stabilität, physikalischen Eigenschaften und Energieanwendungen. Eine detaillierte schematische Skizze der beiden Übersichtsartikel zu Herstellung, Eigenschaften und Anwendung von TiO2 Nanofluide sind in Abb. 3 zu sehen. Diese Übersicht ist aus der Perspektive einer bestimmten Art von Nanofluid organisiert, die als eine der der praktischen Anwendung am nächsten liegenden Art angesehen wird. Und das Hauptziel dieses Papiers ist es, Forschern ein hilfreiches Nachschlagewerk zur Verfügung zu stellen, um das Wissen über den Forschungsstatus von TiO2 . zu aktualisieren Nanofluids und weisen Sie auf die kritischen Herausforderungen und nützliche Empfehlungen für zukünftige Studienrichtungen hin.
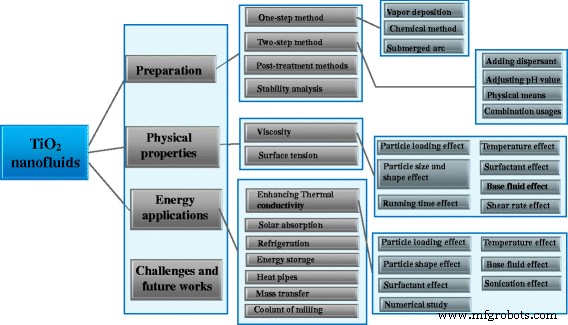
Eine schematische Skizze der Herstellung, Eigenschaften, Anwendungen und Herausforderungen von TiO2 Nanoflüssigkeiten
Vorbereitung von TiO2 Nanoflüssigkeiten
Einstufige Methode
Generell lassen sich zwei Hauptpräparationsmethoden unterscheiden:Einstufen- und Zweistufenverfahren. Ein einstufiges Verfahren wird implementiert, indem Nanopartikel in das erforderliche Arbeitsfluid suspendiert werden, das ihren Erzeugungsprozess begleitet. Einstufige Verfahren lassen sich weiter in physikalische Verfahren und chemische Verfahren unterteilen. Physikalische Verfahren umfassen Aufdampfen, Laserablation und Unterpulver. Chemische Methode bedeutet, Nanofluide durch chemische Reaktion herzustellen. Im Allgemeinen werden die obigen Verfahren als Herstellungsverfahren für trockene Nanopartikel eingeführt. Diese Methoden können jedoch zu einstufigen Vorbereitungsmethoden für Nanoflüssigkeiten aufgerüstet werden, indem die Trockenpartikelsammler durch die entsprechenden Basisflüssigkeitsbehälter ersetzt werden.
Dampfablagerung
Die Gasphasenabscheidung ist eine gängige physikalische Methode bei der Herstellung von Nanoflüssigkeiten. Ein typisches Gerät für diese Methode ist in Abb. 4 zu sehen [67]. Das Schüttgut zur Herstellung von Nanopartikeln wird in einem mit Inertgas gefüllten Niederdruckbehälter erhitzt und verdampft, anschließend wird der Rohmaterialdampf durch den verwirbelten Flüssigkeitsfilm abgekühlt und in den Basisflüssigkeiten abgesetzt. Die Gasphasenabscheidung wird normalerweise bei der Herstellung von Metall-Nanofluiden verwendet, aber dieses Verfahren wird selten für TiO2 . verwendet Nanofluide wegen der hohen Siedetemperatur. Dieses Verfahren kann jedoch verbessert werden, indem eine elektrische Heizung verwendet wird, um eine hohe Temperatur zu erreichen. Leeet al. [68] nutzten eine einstufige Pulsed-Wire-Evaporation (PWE)-Methode, um auf Ethylenglykol (EG) basierende Nanofluide herzustellen, die TiO2 . enthalten Nanopartikel. Sie legten gepulste 25-kV-Spannungen an einen dünnen Draht an und überhitzten ihn, um in wenigen Millisekunden zu Plasma zu verdampfen. Dann wurde das Plasma mit Argon-Sauerstoff interagiert und zu Nanopartikeln kondensiert. Schließlich erhielten sie TiO2 Nanofluids, indem die Nanopartikel direkt mit EG in der Kammerwand in Kontakt kommen.
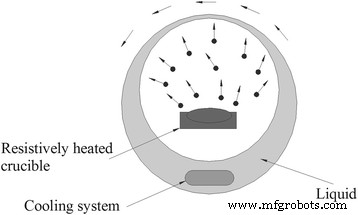
Eine typische Vorrichtung des Aufdampfverfahrens zur Herstellung von Nanofluiden. Neu gezeichnet basierend auf Referenz [67]
Unterwasserbogenmethode
Das Unterpulververfahren kann eine noch höhere Temperatur für die Herstellung von TiO2 . bereitstellen und halten Nanoflüssigkeiten. Changet al. [69] stellten ein neues Unterpulver-Synthesesystem zur Herstellung von TiO2 . her Nanoflüssigkeiten. Ihre Vorrichtung besteht hauptsächlich aus einer Lichtbogensprüheinheit, einem Vakuumraum sowie Temperatur- und Druckkontrollsystemen, die in Abb. 5 gezeigt sind. In dieser Vorrichtung wird TiO2 . in großen Mengen verwendet Feststoff wurde durch das Bogenentladungsverfahren im Vakuum verdampft und dann das gasförmige TiO2 wurde durch eine isolierte Flüssigkeit schnell zu einem feinen Feststoff abgekühlt. Sie kamen zu dem Schluss, dass diese Methode wichtiger ist als Aerosolmethoden, da die hergestellten Nanoflüssigkeiten eine höhere Dispersionsstabilität aufwiesen und als Newtonsche Flüssigkeit angesehen werden könnten. Zhanget al. [70] verbesserten das Unterpulververfahren durch Optimierung des Reaktionsparameterkontrollsystems, des Kühlkreislaufs und der Größe des Unterpulvergeräts. Basierend auf dem optimierten System können sie stabileres und feineres TiO2 . herstellen Suspension mit guter Reproduzierbarkeit der Partikelgröße. Und die Adsorptionsleistung ihres TiO2 Nanopartikel ist besser als kommerzielle.
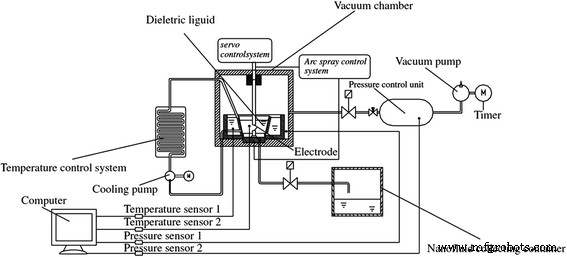
Schematische Abb. des verbesserten Submerged Arc Nanofluid Synthetic System (ISANSS) [40]. Reproduziert mit Genehmigung des Japan Institute of Metals and Materials
Chemische Methode
Ein chemisches Verfahren besteht darin, Nanofluide durch eine chemische Reaktion zu erhalten, und es umfasst im Allgemeinen ein gemeinsames Ausfällungsverfahren und ein Verfahren zur Umwandlung von Vorläufern. Die konventionelle chemische Methode zur Synthese von TiO2 nanofluids basiert auf einer Vorstufe TiO(OH)2 Sediment durch chemische Reaktion von anorganischen Titansalzen und Ammoniak-Wasser, dann kalziniert, um TiO2 . zu erhalten Pulver. Einige Untersuchungen zeigten, dass Nanofluide, die durch das chemische Verfahren hergestellt wurden, eine bessere Stabilität und eine höhere Wärmeleitfähigkeit aufwiesen als solche, die durch das zweistufige Verfahren hergestellt wurden [71]. Die Kontrollierbarkeit der Mikrostruktur von Nanopartikeln ist ein weiteres Unterscheidungsmerkmal dieser Methode. Die konventionelle Einstellmethode ist die Kontrolle der Parameter wie Synthesetemperatur, pH-Wert, Ultraschallbadzeit und der Additive [72]. Dieses Verfahren wird jedoch hauptsächlich verwendet, um TiO2 . herzustellen Pulver durch Trocknen der Flüssigkeit aufgrund der komplexen Flüssigkeitsumgebung bei diesem Verfahren ist für die detaillierte Anwendung von Nanofluiden nicht geeignet. Während das TiO2 Pulver können stabil in der erforderlichen Basisflüssigkeit suspendiert werden, indem die Hauptflüssigkeit ohne Trocknungsprozess gewechselt wird. P>
Das einstufige Verfahren hat die Trocknungs- und Dispergierprozesse nicht enthalten, die anfällig für die Bildung von Agglomerationen von Nanopartikeln sind. Daher wird allgemein angenommen, dass ein einstufiges Verfahren stabilere Nanoflüssigkeiten erhält [73]. Allerdings gibt es auch einige Mängel, die den Anwendungsbereich des Einschrittverfahrens einschränken. Beispielsweise kann Dampfabscheidung nicht verwendet werden, um Nanofluide herzustellen, die hochsiedende oder nichtkristalline Nanopartikel enthalten. Laserablations- und vakuumvergrabene Lichtbogenverfahren sind teuer und erfordern kritische Umstände. Die chemische Methode erfordert im Allgemeinen die Dienste spezifischer Reaktionsbedingungen wie erforderlicher pH-Wert und Temperatur. Und es kann leicht einige Nebenprodukte in den Flüssigkeiten synthetisieren [74]. Zum Beispiel haben Sonawane et al. [75] nutzten die Sol-Gel-Methode zur Synthese von Anatas TiO2 Nanopartikel mit einem konstanten pH-Wert von 5. Die Vorläuferlösung enthielt Titanisopropoxid und Isopropanol sowie bidestilliertes Wasser. Daraus kann geschlossen werden, dass diese Mischung mit solch einem spezifischen pH-Wert und komplexen chemischen Zusammensetzungen nicht als Wärmeübertragungs-Nanofluid verwendet werden konnte. Daher trockneten sie das synthetisierte TiO2 Nanopartikel und redispergieren sie dann mit Ultraschallbehandlungen in die erforderlichen Basisarbeitsflüssigkeiten, einschließlich Wasser, EG und Paraffinöl, um die erforderlichen Nanoflüssigkeiten zu erhalten. Daraus kann geschlossen werden, dass das Ein-Schritt-Verfahren für einige Nanofluide mit spezifischen Inhaltsstoffen, insbesondere für die Nanofluids mit reinem Wasser, Öl, Kältemittel etc. als Basisfluids und auch für ein Anwendungssystem mit flüchtigen Gasen, kaum anwendbar ist.
Zwei-Schritt-Methode
Beim zweistufigen Verfahren werden die Prozesse zur Herstellung von Nanopartikeln und deren Suspendierung in der erforderlichen Basisflüssigkeit unabhängig voneinander betrieben. Zweistufige Methode wird häufig für TiO2 . verwendet Nanofluide seit den Synthesetechniken von TiO2 Nanopartikel haben im Wesentlichen den industriellen Produktionsmaßstab erreicht. Abbildung 6 zeigt ein typisches Verfahren einer zweistufigen Methode. Die trockenen Nanopartikel werden zunächst chemisch oder physikalisch synthetisiert und anschließend in benötigten Basisflüssigkeiten suspendiert. Da jedoch die starke Wechselwirkungskraft der Partikel zu einer Kollision und Aggregation von Nanopartikeln führen kann, ist es für sie ziemlich schwierig, stabil und gleichmäßig in der Basisflüssigkeit zu suspendieren. Daher werden im Allgemeinen einige Dispergierverfahren eingesetzt, um eine gute Stabilität und Verfügbarkeit von Nanofluiden zu gewährleisten.
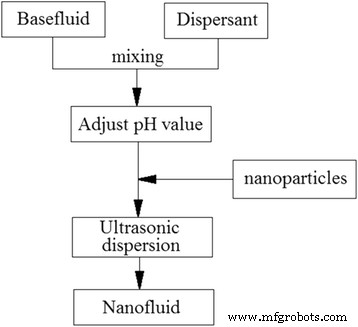
Ein typisches Verfahren der zweistufigen Methode zur Herstellung von Nanoflüssigkeiten [35]. Reproduktion mit Genehmigung von Elsevier
Tabelle 2 zeigt eine Zusammenfassung verwandter Studien zu den Herstellungsmethoden von TiO2 . Nanofluide in den letzten Jahren. Es ist ersichtlich, dass die Arten von Basisflüssigkeiten Wasser, EG, Kältemittel, organische Lösungsmittel usw. umfassten. Im Allgemeinen wurden drei Haupttechniken zum Dispergieren und Suspendieren von Nanopartikeln in Basisflüssigkeiten in dem zweistufigen Herstellungsverfahren weit verbreitet verwendet.
Dispergiermittel hinzufügen
Die erste Dispergiermethode ist die Modifizierung der Partikeloberflächen durch Zugabe von Dispergiermittel, von der erwartet wird, dass sie die Aggregation der Nanopartikel durch die Rolle der elektrostatischen Abstoßung oder sterischen Hinderung der Dispergiermittelmoleküle verhindert [76]. Es ist festzuhalten, dass in den vorliegenden Berichten CTAB das am häufigsten eingesetzte Tensid war. Bei einigen Untersuchungen wurden auch andere Arten wie SDBS, SDS, PVP, Ölsäure, Essigsäure und PEG verwendet. Im Jahr 2012 haben Mo et al. [77] nutzten eine zweistufige Methode, um zwei Arten von Nanofluiden herzustellen, indem stäbchenförmiges Rutil-TiO2 . suspendiert wurde und sphärischer Anatas TiO2 ins Wasser. Sie beobachteten, dass die Nanoflüssigkeiten 286 h lang stabil bleiben können, wenn SDS als Dispergiermittel verwendet wird. Im folgenden Jahr verglichen sie die Auswirkungen von drei verschiedenen Tensiden auf die Dispergierung, darunter SDBS, PVP und CTAB [78]. Und sie fanden heraus, dass sie in diesem experimentellen Forschungsbereich, wenn das Massenverhältnis der SDBS und der Titandioxid-Nanopartikel 0,3 beträgt, die beste Dispersion des Nanofluids erhalten können. Nakayama und Hayashi [79] verwendeten ein zweistufiges Verfahren, um eine hohe Beladung mit TiO2 . zu dispergieren Nanopartikel in einer organischen Basisflüssigkeit mit Hilfe der Oberflächenmodifizierung durch Propionsäure und n-Hexylamin. Sie fanden heraus, dass die Oberflächenmodifizierung die Dispersion von Nanofluiden verbessern kann, was bei der Zweistufenmethode eine bessere Wirkung zeigte als bei der Einstufenmethode. Die Eigenschaften von TiO2 Die von ihnen hergestellten Nanopartikel werden nicht verändert und können gut für verschiedene Flüssigkeiten auf organischer Lösungsmittelbasis verwendet werden.
pH-Wert anpassen
Das zweite Dispersionsverfahren besteht darin, die Dispersionsumgebung durch Einstellen des pH-Werts der Basisflüssigkeit einzustellen. Diese Methode besteht darin, den Nanopartikeln ein höheres Zeta-Potential auszustatten, indem ein geeigneter pH-Wert der Flüssigkeit eingestellt wird, wodurch erwartet wird, dass die Nanopartikel durch die höhere elektrostatische Abstoßung kontaktiert werden [76]. Li und Sun [80] untersuchten den Einfluss des pH-Werts auf das Aggregationsverhalten von TiO2 Nanopartikel in mono- und binären basischen Flüssigkeiten durch SRFA und Fe(III). Sie fanden heraus, dass die Adsorption von SRFA die Suspensionsstabilität von TiO2 . stark verbesserte Nanopartikel bei pH-Werten von 4, 6 und 8, und sie dachten, dass dies hauptsächlich durch den starken Anstieg der negativen Ladungen auf der Oberfläche der Partikel verursacht wird. Er et al. [81] fanden heraus, dass die Stabilität von TiO2 Nanofluide können stark verbessert werden, indem der pH-Wert der Basisflüssigkeit auf 11 eingestellt wird, bei dem ein hohes Zetapotential von 45 mV gebildet werden kann, um eine erneute Agglomeration und Ablagerung und eine mögliche anschließende Verschmutzung des Kupferrohrs zu verhindern. Die Nanofluide mit dem optimalen pH-Wert können mehrere Monate stabil bleiben. Auch Vakili et al. [82] und Sen et al. [83] stellten den pH-Wert der Basisflüssigkeit auf 11 ein und fanden heraus, dass das TiO2 Nanofluide können unter diesen stark alkalischen Bedingungen eine bessere Dispersionsstabilität aufweisen.
Physische Mittel
Das dritte Dispergierverfahren ist gleichbedeutend mit dem Aufbrechen von Partikelagglomerationen durch physikalische Mittel, beispielsweise mechanisches Rühren, Ultraschallwellen und Rührkugelmahlen. Diese Verfahren sollen Kavitationsschwingungen erzeugen, die zu Scher-, Bruch- und Streueffekten führen können [84]. Es ist allgemein anerkannt und bewiesen, dass die Nanofluide nach richtiger Überschallvibration stabiler sind und dies kann erneut durch die Zusammenfassung der Dispersionsstabilität TiO2 . bewiesen werden Nanoflüssigkeiten. Aus Tabelle 2 ist ersichtlich, dass fast alle Herstellungsverfahren einige physikalische Behandlungen umfassten. Liet al. [85] dispergiertes TiO2 Nanopartikel in MDEA-Lösung zur Herstellung von TiO2 –MEDA–H2 O Nanoflüssigkeiten. Sie fanden heraus, dass die Nanoflüssigkeiten bei mechanischer Bewegung ohne Zugabe von Dispergiermittel 48 Stunden lang stabil bleiben konnten. Tadschik et al. [86] untersuchten die Auswirkungen verschiedener Ultraschalltypen (kontinuierliche oder unterbrochene Pulse) auf das Suspendierungsverhalten von wasserbasiertem TiO2 Nanoflüssigkeiten. Die Ergebnisse zeigten, dass die kontinuierlichen Pulse bessere Brechwirkungen aufwiesen als die diskontinuierlichen, während letztere einige große Aggregate nicht trennen konnten. Silambarasanet al. [66] untersuchten experimentell die Wirkung von Rührwerkskugelmahlen und Ultraschall auf das Suspendierverhalten von wasserbasierten Gemischen, die Submikron-TiO2 . enthielten Partikel. Sie fanden heraus, dass Rührkugelmahlen stabile Suspensionen mit Submikron-Partikeln erzeugen kann und Ultraschall weiter angewendet werden kann, um das Transportverhalten des TiO2 . zu kontrollieren Suspensionen. Longo und Zilio [87] verglichen die Auswirkungen von mechanischem Rühren und Ultraschallwellen auf das Dispersionsverhalten von TiO2 –Wasser und Al2 O3 –Wasser-Nanoflüssigkeiten. Sie beobachteten, dass die Behandlung mit Ultraschall bei 25 kHz für 48 Stunden eine bessere Dispersionseffizienz zeigte als nur einfaches mechanisches Rühren. Nach diesen physikalischen Dispersionsbehandlungen können beide Arten von Nanoflüssigkeiten länger als 1 Monat stabil bleiben.
Kombinierte Verwendungen
Im Allgemeinen werden Kombinationen von Dispergierverfahren der Zugabe von Tensid, Änderung des pH-Werts von Basisflüssigkeiten und Ultraschallvibration in zweistufigen Verfahren verwendet, um eine bessere Dispergierleistung von Nanoflüssigkeiten zu erzielen. Liuet al. [88] dispergiertes TiO2 Nanopartikel (25 nm) in Wasser zur Herstellung von stabilem TiO2 Nanoflüssigkeiten. Drei Behandlungen einschließlich Zugabe von PEG1000 als Dispergiermittel, Ultraschallvibration und Regulierung des pH-Werts auf 4–5 oder 9–10 wurden verwendet, um stabiles TiO2 . zu erhalten Nanoflüssigkeiten. Fedeleet al. [89] verwendeten ein kombiniertes Dispergierverfahren aus Zugabe von Essigsäure als Dispergiermittel und Einstellung des pH-Werts auf einen Bereich von 1,86 bis 3,07 entsprechend den Massenanteilen der Nanopartikel sowie einer geeigneten Beschallung; Sie beobachteten, dass die Nanoflüssigkeiten mindestens 35 Tage lang stabil bleiben konnten, da die durchschnittliche Partikelgröße während der Zeiträume ungefähr konstant blieb. Ghadimiet al. [90] stellten ein extrem stabiles wasserbasiertes TiO2 . her Nanofluid durch Zugabe von Essigsäure und Einstellen des pH-Werts auf 5 sowie Ultraschallvibration. Sie fanden das TiO2 Nanoflüssigkeiten waren nach 1 Jahr Lagerung immer noch stabil suspendiert. Es gibt auch einige andere Beispiele für die kombinierte Anwendung der drei Techniken. Aus Tabelle 2 ist ersichtlich, dass Mo et al. [77, 78], Kim et al. [91], Mushed et al. [92], Jarahnejad et al. [93], Ghadimi et al. [90] und Said et al. [94] nutzte alle drei Dispersionstechniken, um den besten Dispersionseffekt zu erzielen.
Ein sich ändernder pH-Wert von Basisflüssigkeiten schränkt jedoch den Anwendungsbereich des TiO2 . stark ein Nanofluide als Thermofluide aufgrund der Korrosion und Sicherheit in sauren und alkalischen Bedingungen. Daher neigen mehr Forscher eher dazu, die anderen beiden Dispersionstechniken zu verwenden, nämlich. Zugabe von Dispergiermittel und physikalischen Mitteln für die potentiellen Anwendungen in tatsächlichen Systemen. Wuet al. [95] und Yang et al. [74] soll TiO2 . auftragen Nanofluids zu Ammoniak-Wasser-Absorptionskältesystem. Die Methode zur Änderung des pH-Wertes steht nicht zur Verfügung, da die Basisflüssigkeit einen bestimmten pH-Bereich hat, der durch die Ammoniakkonzentration bestimmt wird. Daher verwendeten sie PAA oder PEG1000 als Dispergiermittel in Kombination mit Ultraschallschwingungen, um die Stabilität von TiO2 . zu verbessern Nanofluids und erzielten gute Effekte. Um Nanoflüssigkeiten auf Kompressionskältesysteme anzuwenden, haben Peng et al. [96] TiO2 . hinzugefügt Nanopartikel in R141b, um ein Nano-Kältemittel mit einer Partikelgröße von 25, 40, 60 bzw. 100 nm herzustellen. Das Nano-Kältemittel wurde mit einem Ultraschallprozessor 20 Minuten lang beschallt. Und sie hielten diesen Schritt für wichtig, um eine gute Dispergierung von Nanopartikeln in Kältemittelmassen zu erreichen. Außerdem untersuchten sie experimentell den Einfluss anionischer, kationischer und nichtionischer Tenside auf die Stabilität von Nano-Kältemitteln. Und sie beobachteten, dass der Tensidtyp ein wichtiger Faktor für die stationäre Partikelgröße ist. Kayhaniet al. [97] verwendeten Tensid Hexamethyldisilazan und Ultraschall-Vibrationsmethoden hergestelltes trockenes TiO2 Nanopartikel zuerst und dann in destilliertes Wasser mit Ultraschallvibrationsbehandlung (400 W und 24 kHz) für 3–5 h gegeben. Sie fanden heraus, dass die hergestellten Nanoflüssigkeiten mehrere Tage stabil bleiben konnten und keine Sedimentation auftrat. Yanget al. [98] fanden heraus, dass die Verwendung von Tensid SDBS in einem niedrigen Konzentrationsbereich und Ultraschallvibration das Suspendierverhalten von auf Ammoniak-Wasser basierendem TiO2 . verbessern kann Nanoflüssigkeiten.
Nachbehandlungsmethoden
Neben konventionellen ein- oder zweistufigen Verfahren wurden auch einige Nachbehandlungsmethoden zur Herstellung von Nanofluiden vorgeschlagen. Einige besser dispergierte Nanoflüssigkeiten können aus einigen schlecht dispergierten Rohflüssigkeiten, die agglomerierte Nanopartikel enthalten, durch einige spezielle Behandlungen erhalten werden, z. B. durch Aufbrechen oder Entfernen der agglomerierten Nanopartikel aus der Rohflüssigkeit.
Hwanget al. [99] beobachteten, dass die Wirkung von Rührer, Ultraschallbad und Ultraschall-Disruptor zur Verbesserung der Dispergierung von Nanofluiden begrenzt ist. Sie verwendeten einen Hochdruckhomogenisator, um das Nanofluid zurückzuziehen, und der Prozess ist in Abb. 7 zu sehen. In ihrer Forschung konnte der anfängliche durchschnittliche Durchmesser der Partikel nach der erneuten Behandlung um mindestens eine Größenordnung verringert werden der Hochdruckhomogenisator. Und sie fanden heraus, dass der Hochdruckhomogenisator die beste Wirkung unter allen in ihrer Studie verwendeten physikalischen Dispergiermitteln zeigte.
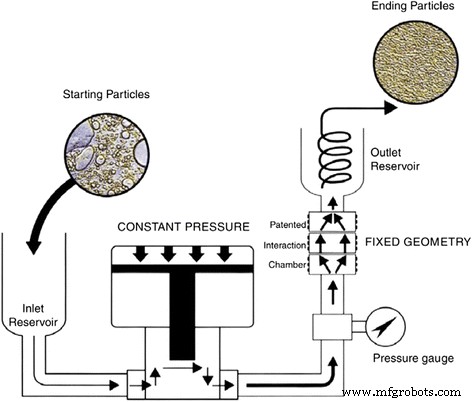
Schematische Darstellung des Hochdruckhomogenisators zur Herstellung von Nanofluiden [99]. Reproduziert mit Genehmigung von Elsevier
Yanget al. [100] verwendeten eine Optimierungsmethode zur Herstellung von Nanofluiden. Der Optimierungsprozess der Dispersionsverbesserung von Nanofluiden ist in Abb. 8 dargestellt. Sie entfernten die gut suspendierten Nanofluide aus den höher konzentrierten Nanofluiden und brachten dann die entfernten Teile durch Verdünnung der Zugabe von Basisfluiden in die erforderlichen Konzentrationen zurück. Das Verdünnungsverhältnis basierte auf der Eigenschaft, dass die Absorptionsfähigkeit der Nanoflüssigkeiten direkt proportional zu ihrer Konzentration ist. Und sie beobachteten Sedimentationen und maßen die Variation der Saugfähigkeit, um die Wirkung der Methode abzuschätzen. Die Ergebnisse zeigten, dass sowohl für Rutil als auch für Anatas TiO2 Nanofluids kann das optimierte Verfahren deren Dispersion stark verbessern und stabileres TiO2 . produzieren Nanoflüssigkeiten.
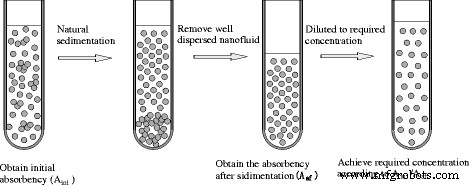
Optimierungsprozess der Dispersionsverbesserung von Nanofluiden [132]. Reproduziert mit freundlicher Genehmigung von Taylor &Francis
Es gibt einige Kontroversen oder Inkonsistenzen in der Argumentation der Herstellung von Nanofluiden. Erstens ist es eine Inkonsistenz, ob man das Ein-Schritt-Verfahren oder das Zwei-Schritt-Verfahren anwendet. Es wird erwartet, dass ein einstufiges Verfahren eine bessere Dispersionsstabilität erreicht, da es die Trocknungs- und Dispergierprozesse von Nanopartikeln vermeidet. Für die Nebenwirkungen der einstufigen Methode wie Nebenprodukt erscheinen jedoch spezielle Lösungsumgebungen fataler, was den Anwendungsbereich von Nanofluiden stark einschränkt. Daher wird aufgrund der großen Anpassungsfähigkeit und erheblichen Verbesserung der Dispersionstechniken von TiO2 . das zweistufige Verfahren häufiger verwendet Nanopartikel. Insgesamt wird empfohlen, ein zweistufiges Verfahren mit entsprechender Nachbehandlung zur Herstellung von TiO2 . anzuwenden Nanoflüssigkeiten.
Eine weitere Kontroverse ist, ob Tenside bei der Herstellung von Nanofluiden verwendet werden sollten. The presence of appropriate surfactant can improve the dispersion stability but also may bring some side effects such as a decrease in thermal conductivity, increases in viscosity, and foaming ability. Due to the potential advantages such as reduction in surface tension and improvement in re-dispersible property, the surfactant with low concentration is suggested to be used when it not brings obvious decrease in thermal conductivity or increase in viscosity and foaming ability. In addition, the influence of surfactant on thermal conductivity and viscosity of nanofluids is also a controversy in current studies.
Stability of Nanofluids
Stability research is generally followed the preparation to achieve the optimal dispersion craft since it is closely related to the effectiveness and practicability of nanofluids. The great amount of aggregations in the unstable nanofluids can easily cause sedimentation and adsorption on the inner surface of the system, which will probably result in the degradation of heat transfer efficiency, raising of pumping power, and even blocking up in system pipe blocks.
It can be found from Table 2 that the stable times of different researchers thought were variously distributed in the range of several hours to 1 year. A most stable nanofluid was obtained by a combined use of adding surfactant, controlling pH value, and ultrasonic vibration by Ghadimi et al. [90]. Also, the particles’ loading in their experiment was very low at 0.1 wt.%, which was also another contribution for the long-term stability. Without adding surfactant, the nanofluids can also achieve a better dispersion stability by adjusting the pH value of the liquid to a suitable value. For example, He et al. [81] and Longo et al. [87] observed that the TiO2 nanofluids can keep stable for months by adjusting the pH to 11 with the help of ultrasonic vibration. Also, some TiO2 nanofluids with good dispersion stability were prepared only through physical means in some research. Padmanabhan et al. [101] used a magnetic stirring to prepare R134a and mineral oil-based TiO2 nanofluids that can keep stable for 6 months. This is likely because the particles’ loading employed in their study is very low (0.1 g/L) and the high viscosity of the R134a and mineral oil base fluid can also provide a superior dispersion condition. This conclusion can also serve as proved by Palabiyik et al.’s results [102]. They obtained a TiO2 nanofluids stable for several months by the help of sonication with a higher viscosity propylene glycol as base fluid. The similarity is that they were both using organic solvent of high viscosity as base fluids and the best ones was only treated by physical means. Also, it can be seen that TiO2 nanoparticles have a comprehensive dispersivity in both polar aqueous solution and nonpolar organic solution.
However, the above judgments on dispersion stability of various TiO2 nanofluids are not very objective and accurate because most of the results showed the least stable time. Moreover, there is no uniform standard for evaluating the stability of nanofluids, and the stability evaluating methods in different research were sufficiently different. Current evaluation methods of stability of nanofluids mostly consisted of observing the stratification or sedimentation and testing the zeta potential, particles’ size, or absorbency. Mansel et al. [103] used the sedimentation observation method and zeta potential method to evaluate the stability of TiO2 –water nanofluids in different pH values. They observed that in low or high pH value, the TiO2 –water nanofluids can obtain good stability. Mo et al. [78] used zeta potential method to investigate the stability of TiO2 –water nanofluids with three different surfactants SDBS, PVP, and CTAB, respectively. By comparing the value of zeta potential, they obtained the optimal kind of surfactant and the best dispersion of nanofluid. Wei et al. [104] used sedimentation, zeta potential (ζ), and size analysis to evaluate the stability of diathermic oil-based TiO2 nanofluids. They found that there was not obvious sedimentation and the zeta potential (ζ) and size analysis also showed good results. They thought the TiO2 nanofluids they prepared were very stable and can be used to enhance heat transfer for a fluid system. Li et al. [105] used sedimentation observation to investigate the stability of TiO2 –MDEA–water nanofluids. They found that after a specific period of mechanical agitation, the sedimentation was reduced and the stability of nanofluids was improved. However, the ultrasonic vibration will deteriorate the stability of TiO2 –MDEA–water nanofluids. For this reason, only mechanical agitation was employed in their research. Yanget al. [74] investigated the dispersion behavior of 20 types of nanoparticles in binary base fluid of ammonia–water by measuring the absorbency of nanofluids, and they defined ratio of varying absorbency to quantitatively compare the suspending stability of different kinds of nanoparticles, dispersant, and base fluid mixtures. They observed that the new defined index was more applicable than conventional means because it could directly compare the suspending behavior of various kinds of nanofluids. While the method of observing the stratification or sedimentation is restricted for nanofluids in different colors or without distinctly stratification after standing. The results showed that the anatase and rutile TiO2 nanofluid were the most stable metal oxides without any surfactant. And when adding optimal dispersant, anatase TiO2 nanofluid was still the most stable one.
Generally, the combination of several stability evaluating methods is employed to investigate the stability of nanofluids more accurately. Silambarasan et al. [66] used method of measuring the particle size distribution, zeta potential, and microscopy of grain size methods to characterize the suspending stability of TiO2 nanofluids. By those methods, they prepared remarkably stable TiO2 nanofluids whose absorbency changed very little after 10 days. Tajik et al. [86] used sedimentation observation and microscopy of grain size to investigate the roles of ultrasonic wave types on the suspending behavior of nanofluids. And they found that the pulses in discontinues type could not smash some big clusters or aggregations since the sedimentation occurred after 48 h of storage.
Physical Properties of TiO2 Nanofluids
The physical properties of TiO2 nanofluids are focused on the viscosity and thermal conductivity. Also, a few papers investigated the surface tension. Using nanofluids to enhance the thermal conductivity is a typical application in heat transfer filed. Therefore, the thermal conductivity of TiO2 nanofluids will be introduced in part 2 of the reviews. In part 1, the viscosity and surface tension are introduced as follows.
Viscosity
Viscosity is an essential parameter for nanofluids especially for flow and heat transfer applications because both the pressure drop and the resulting pumping power are depended on the viscosity. Viscosity describes the internal resistance of a fluid to flow, and it is an important property for all thermal and flow applications for nanofluids. The nanofluids with higher viscosity will result in higher flow resistance and lower flow velocity, which also induce the decrease of the heat transfer. To obtain flow velocity and heat transfer efficiency, more pumping powers are needed which induce more energy consumption. Moreover, for some mass transfer application of nanofluids, viscosity plays more important roles than thermal conductivity because the viscosity determines the mass transfer resistance of molecules entering the liquid surface and the diffusion coefficient in the liquid. Murshed and Estellé [106] provide a state-of-the-art review on the viscosity of various nanofluids. They found that the experimental data from various literatures are greatly scattered and not consistent even for the same nanofluids. This review will discuss in detail the influence factors on the viscosity of TiO2 nanofluids to provide an exhaustive knowledge on this topic.
Particle Loading Effect
Many literatures have concerned the volume concentration effect on the viscosity of TiO2 nanofluids. Table 3 shows the particle loading dependence of the viscosity of TiO2 nanofluids in different research. It can be observed that the viscosity of the TiO2 nanofluids increases with the increase of the particle loading. However, some works showed that the viscosity ratio varies linearly with variation of volume concentration, but some other results showed the viscosity ratio variation is parabolic. The viscosity enhancements of TiO2 nanofluids were greatly distinguishing in various researches. For example, in Vakili et al. [82], Arulprakasajothi et al. [107], Duangthongsuk and Wongwises [108], Saleh et al. [109], and Mahbubul et al.’s [110] results, the increments of viscosity were below ten times of the volume percentage of the added particles. However, He et al. [111] and Turgut et al.’s [112] results showed that the viscosities were increased by more than 100 times of the volume percentage of the TiO2 particles added. There are also many results distributed between the values in the above two extreme cases. Therefore, it can be concluded that the influence of particle loading on the viscosity of TiO2 nanofluids is more complex than that on thermal conductivity due to the widespread data in various studies.
Temperature Effect
Besides the volume concentration effect, the temperature effect on the viscosity of TiO2 nanofluids is also widely studied by many researchers. He et al. [111] prepared four different concentration TiO2 –H2 O nanofluids with 20 nm TiO2 and measured the viscosities of TiO2 –H2 O nanofluids and deionized water with different temperatures. They observed that the TiO2 –H2 O nanofluids were Newtonian fluids, which were the same as Chang and Liu’s finding [69], and the viscosities varied inversely with the temperature of the TiO2 –H2 O mixture system. Ling et al. [113] also measured the viscosities of the TiO2 –H2 O nanofluids with different mass fractions, when temperature varied from 15 to 40 °C. They found that the viscosity of the nanofluids increased when fluids thicken and decreased with the increment of the temperature exponentially. Liuet al. [114] figured that the viscosities of TiO2 –H2 O nanofluids increase remarkably with the volume fraction of nanoparticles and vary oppositely to the temperature of the TiO2 –H2 O nanofluids greatly with similar experimental method. Based on the value of the viscosities, they also propose an amended suspension viscosity formula. Some research results showed that the viscosity of nanofluids is a function of volume loading and temperature as well as base fluid viscosity. Yiamsawas et al. [115] measured the viscosity of TiO2 –water with a volume loading varied from 1 to 8% at a high-temperature range of 15 to 60 °C. By comparisons with previous studies, they proposed a useful correlation for practical applications which indicated that the viscosity of nanofluids is a function of volume loading and temperature as well as the base fluid’s viscosity.
Comparing with the absolute viscosity, the varieties of relative viscosity at different temperatures were more impressive for researchers. Jarahnejad et al. [93] carried out a detailed study on the effect of temperature on the viscosity and the relative viscosity of TiO2 respectively. And the results are shown in Fig. 9. It can be found that compared to base water, the average viscosities of TiO2 nanofluids increased by 17, 50, and 78% for 3, 6, and 9 wt.% of particles’ loading, respectively, at 20 °C. The viscosity of nanofluids with different particle loading decreased as the temperature increased, while the relative viscosity remained nearly constant with the temperature. The observation of independent of temperature can be also included in some other research. Fedele et al. [89] presented the characterization of water-based nanofluids where TiO2 ranging between 1 and 35% in mass. They concluded that the relative viscosity was independent from temperature for all the particle loading employed. And the nanofluids at 1 wt.% exhibited a water-like behavior within the experimental error. But this observation was invalid at the higher concentrations (+243% for 35 wt.% at 343 K). Also, Silambarasan et al. [66] found that the temperature has a smaller effect on the relative viscosity since the viscosity of TiO2 suspensions was reproducible even after repeated and alternating heating and cooling processes. And they attributed the reason to the effect of particles’ temperature-dependent intermolecular forces in the suspension. However, some different results can also be observed. Teng et al. [116] found that the relative viscosity increased from 8.2 to 16% when the temperature varied from 10 to 40 °C for the TiO2 nanofluids with 0.5 wt.% of particle loading. Cieśliński et al. [117] found that the relative viscosity of thermal oil-based TiO2 nanofluids remained constant when the temperature varied from 20 to 40 °C, but had a nearly linear increase with the increase of temperature when exceeding 40 °C. Yapici et al. [118] observed that the effect temperature was different for different shear rate. The relative viscosity measured was independent of the temperature at a higher shear rate region. However, for lower shear rate region, a great temperature dependency behavior of viscosity of TiO2 nanofluids was exhibited especially at high temperatures
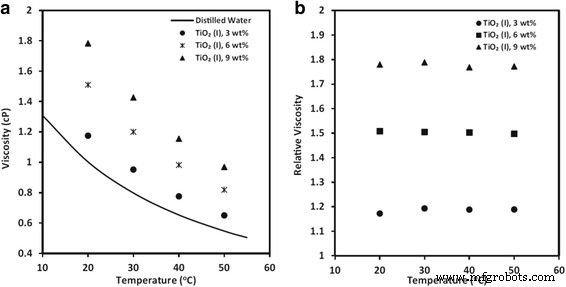
Dynamic viscosity (a ) and relative viscosity (b ) for TiO2 water-based nanofluids at different temperatures [93]. Reproduced with permission from Springer
Particle Size and Shape Effect
The particle size and shape effects on the viscosity of TiO2 nanofluids were not investigated as widely as that of particles’ loading or temperature. In particular, Chen et al. [64, 119] investigated experimentally the viscosity of spherical (25 nm) and rod-like (10 × 100) TiO2 nanoparticle-based nanofluids with water and EG as base fluid, respectively. They found that the viscosity of TiO2 nanofluids was more sensitive to the rod-like particles than spherical particles. It can be seen from Table 3 that the viscosity was increased by 0.5–23% when adding 0.1–1.86 vol.% of spherical TiO2 nanoparticles, while increased by 1–82% when adding 0.1–0.6 vol.% of rod-like TiO2 Nanopartikel. The same observation can also be found for EG-based nanofluids.
Surfactant Effect
The surfactants have been observed to have great effects on the viscosity of TiO2 nanofluids in some recent research. Jarahnejad et al. [93] investigated the effect of two kinds of surfactant trioxadecane acid and poly carboxylate on the viscosity of TiO2 nanofluids respectively. Their results of the dynamic viscosity of 9 wt.% TiO2 –water nanofluids with different surfactants vs. temperature are shown in Fig. 10. The results demonstrated only a very slight increase was found in the viscosity of nanofluids even with the highest particle loading viz. 9 wt.%. However, the two kinds of surfactants could greatly increase the viscosity of nanofluids in the temperature range of 20–50 °C, especially for trioxadecane acid. The similar effect of surfactant on viscosity can also be observed in Ghadimi and Metselaar’s report [90], in which they found SDS can also increase the viscosity of TiO2 nanofluids with 0.1 wt.% particle loading. It was also observed there were important roles of SDS in the long-term dispersion stability of TiO2 nanofluids. Therefore, they still suggested that the dispersion method of adding surfactant and ultrasonic vibration to be adopted in the preparation of nanofluids.
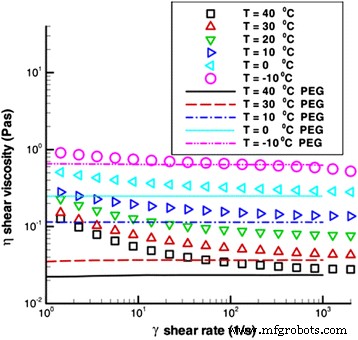
Dynamic viscosity of 9 wt.% TiO2 –water nanofluids with different surfactants vs. temperature [93]. Reproduced with permission from Springer
However, the above results cannot prove that all kinds of surfactant will result in high viscosity for nanofluids. Figure 11 shows the viscosity of TiO2 nanofluids with PEG600 as surfactant measured by Bobbo et al. [120]. It can be seen that the viscosity of base water will not increase but decrease slightly when adding PEG600 at 0.02 or 0.2% loadings. Also, the viscosity of nanofluid containing 0.01% TiO2 nanoparticles and 0.02% PEG600 was a little lower than that of the base water. However, for higher loading of PEG, the viscosity will be greatly increased whether or not containing nanoparticles. It can be seen from Fig. 11 that the nanofluids containing 2% PEG600 and 1% TiO2 nanoparticles showed a viscosity higher than 7% in respect to water, which was analogous at each temperature. The above observation showed the viscosity of nanofluids can be lower than the base fluid in some cases, which also occurred in SWCNT nanofluids in their experiment. The decline of viscosity of fluid when adding surfactant or nanoparticles was also been found in some other research. Yanget al. [121] found that emulsifier OP-10 can reduce the viscosity of ammonia–water in lower concentrations. Ling et al. [122] observed that adding SDBS or OP-10 in TiO2 nanofluids with a lower loading can induce a slight drop in viscosity. Therefore, it is an important issue to choose the suitable surfactants to improve the dispersion stability without increasing the viscosity significantly.
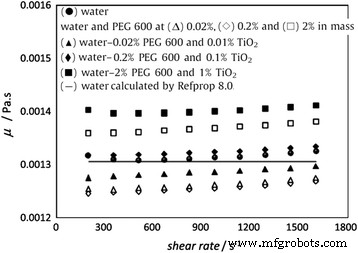
The viscosity of TiO2 nanofluids with PEG600 as surfactant [120]. Reproduced with permission from Elsevier
Base Fluid Effect
The information about base fluid effect on viscosity can be illuminated though Chen et al.’s study [119], in which they found the relative increments of viscosity of water-based TiO2 nanofluids were distinctly higher than that of EG based. It seemed that the higher viscosity the base fluid could result in lower increment in viscosity. Mahbubul et al. [110] found that the viscosity of R123 was increased by only 5.2% when adding 2 vol.% TiO2 Nanopartikel. Sen et al. [78] and Yapici et al. [118] found relative increments of viscosity about 20 times of the particles’ volume percentages. It also seems that TiO2 nanoparticles are more suitable in the organic liquid because a lower relative increment in viscosity can be obtained especially at the higher temperature. Yiamsawas et al. [123] conducted experiments on a mixture with TiO2 nanoparticles and EG/water (20/80 wt.%) in which the volume loading ranged from 0 to 4% and temperature ranged from 15 and 60 °C. They used the experimental data to present a useful correlation to predict the viscosity.
Shear Rate Effect
Another main distinction on the viscosity of TiO2 nanofluids in different research is that whether the fluids were Newtonian fluids in different shear rates. A typical Newtonian nanofluid can be found in foregoing Fig. 11. However, it can be observed from Table 3 that more than half of the results showed that the TiO2 nanofluids in their work are Newtonian fluids, but some others come to the opposite conclusion. Research on rheological characteristic has demonstrated that whether or not the TiO2 nanofluids exhibit Newtonian behavior is also affected by other factors, including the base fluid type, temperature, and particle loading. A quintessential example can be found in Chen et al.’s research [64], where they measured the viscosity of four types of nanofluids made of TiO2 nanoparticles (25 nm) and TiO2 nanotubes (10 nm × 100 nm) dispersed in water and EG. They found that EG–TiO2 nanofluids exhibited Newtonian behavior, whereas water–TiO2 , water–TNT, and EG–TNT nanofluids exhibited non-Newtonian behavior. They indicated that the rheology behavior of TiO2 nanofluids is affected by their specific ingredient and environment, such as particles’ shape and liquid circumstance. The rheological characteristic of TiO2 nanofluids is also related to the temperature. Yapici et al. [118] investigated the rheological characteristic of 9 wt.% TiO2 –water nanofluids with different surfactants vs. temperature. The results are shown in Fig. 12. It can be observed that the base fluid PEG was a typical Newtonian fluid in all kinds of temperature. However, TiO2 –PEG200 nanofluids were nearly Newtonian fluid at a lower temperature and higher shear rate, but it changed into non-Newtonian fluid at higher temperature and lower shear rates. Also, in Said et al.’s results [94], the TiO2 nanofluid with 0.1 vol.% loading was Newtonian fluid at 55 °C, whereas it was non-Newtonian below this temperature for 0.3 vol.% particle loading.
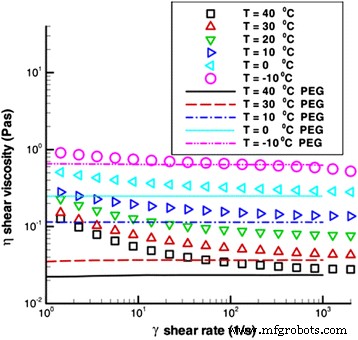
Shear rate dependency of viscosity as a function of temperature for 5 wt.% TiO2 –PEG200 nanofluids [118]. Reproduced with permission from Springer
Running Time Effect
When the nanofluids are actually used in a running system, the time-dependent properties of nanofluids should be a crucial issue for the sustainable application. However, this matter has not been widely studied because of the faultiness in the development of nanofluids. It is generally considered that the thermal and rheological properties of nanofluids will be deteriorated due to the aggregation of nanoparticles after running a long time in the system. However, an opposite result in the time-dependent viscosity of TiO2 nanofluids can be observed in Said et al.’s research [94]. Their results for viscosity of TiO2 –water nanofluid with different volume loading and temperature as well as running time are shown in Fig. 13. It can be observed that the viscosity of fresh samples and the stale samples after running in a flat plate solar collector for 1 month were distinctly different. The viscosity of TiO2 nanofluids was decreased after undergoing the alternative variations in temperature and flow rate in the cycle. This observation was quite interesting and could not be explained anywhere else in the literature. They thought this finding could open new research scope for the applications of nanofluids for a long-term use.
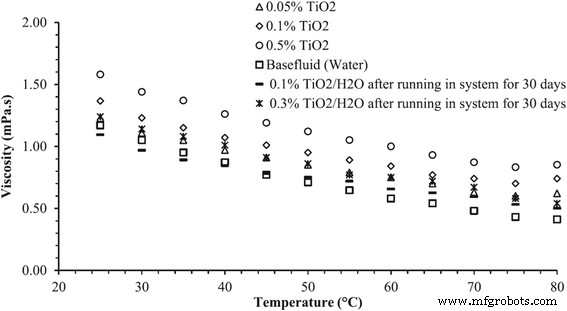
Viscosity of TiO2 –water nanofluid with different volume concentrations and different temperatures [94]. Reproduced with permission from Elsevier
An inconsistency in viscosity of TiO2 nanofluids is quite evident. The intensities of growth in viscosity of TiO2 nanofluids with particle loadings greatly differ in various studies. And there is not yet a universal agreement on the effect of temperature, base fluid, and surfactant on viscosity of TiO2 nanofluids. Moreover, the biggest controversy on viscosity of nanofluid is that whether nanofluid is Newtonian fluid or not. The results in Table 3 exhibit that a substantial part of TiO2 nanofluids in their work are Newtonian fluids, but also, some others exhibit non-Newtonian behavior. The pronounced differences in different samples are mainly due to the complex influence factors on the rheological property. The shear rate has been proved to have great effect on the rheological property, and also, it has combined effect with other factors including temperature, shearing time, particle loading, base fluid type, and particle shape [124], which make it rather difficult to predict whether a nanofluid is Newtonian fluid or not except by experimental means.
Surface Tension of Nanofluids
The research on surface tension of TiO2 –H2 O nanofluids is much less than that of thermal conductivity or viscosity. Some results showed that adding TiO2 nanoparticles had little effect on the surface tension of nanofluids. Liuet al. [125] prepared TiO2 –H2 O nanofluids whose particle size ranged from 11 to 50 nm and the surface tensions TiO2 –H2 O nanofluids were investigated experimentally. They found the surface tension had no obvious change with the increase in particle loading because the surface tension of nanofluids (1% mass fraction) increased only 1.6% compared with deionized water. Hu et al. [126] found the surface tension of TiO2 –H2 O nanofluids increases slightly when adding nanoparticles. And the surface tension decreased as an increase in temperature. Buschmann and Franzke [127] found that no obvious variation occurs when adding a high-volume fraction (5 vol.%) of TiO2 nanoparticles in water. Tian and Wang [128] measured the surface tension of TiO2 –water nanofluids by Jolly balance and abruption method. They found that the surface tension behavior of TiO2 –water nanofluid was the same as water viz. the surface tension decreased as the temperature increases. However, the variation of surface tension is related to the content of nanoparticles. When the content of nanoparticles increases rapidly, the decrease rate of surface tension of TiO2 –water nanofluids will slow down. Yanget al. [129] observed that nanoparticles have little effect but the surfactant can greatly change the surface tension of nanofluids, when the loading of surfactant is below the critical micelle concentration (CMC). And they explained this appearance as follows:The effect of surfactant on the surface tension of liquid is much greater than that of nanoparticles. When adding nanoparticles into a fluid containing surfactant whose loading is below CMC, the “free” surfactant will be absorbed on the surface of nanoparticles and then immersed in the liquid, which can weaken the reducing effect of surfactant on the surface tension of liquids.
However, some results also revealed that the nanoparticles played an indispensable role in the surface tension of nanofluids. Chinnam et al. [130] measured the surface tensions of Al2 O3 , ZnO, TiO2 , and SiO2 nanofluids with a mixture of 60% propylene glycol and 40% water as base fluids, respectively. They only used one average particle size of 15 nm for TiO2 nanofluid due to limiting of manufacturer. They presented a single correlation as a function of volume loading and particle size as well as temperature for all the nanofluids by statistical analysis based on the experimental results. The experimental and fitting results related to TiO2 nanofluids are shown in Fig. 14. It was observed that the surface tension of nanofluids decreased as the temperature and particle volume loading increase and the correlation perfectly fitted the experimental data. In addition, they also observed that the surface tension decreased as the particle size decrease for a certain loading and temperature of nanofluids except the ZnO nanofluid.
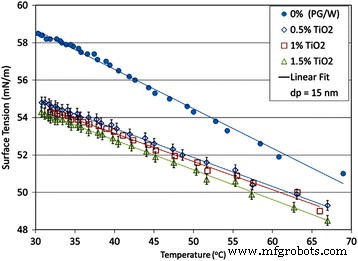
Variation of measured surface tension values of the TiO2 nanofluids with temperature [130]. For different volumetric concentrations up to 1.5% and containing 15 nm particles. Reproduced with permission from Elsevier
Although the surface tension study of nanofluid is not as prevalent as studies in thermal conductivity or viscosity, surface tension is also an important parameter which can affect the film flow especially the initial infiltration of film and the probability of forming channel flow. Due to the effect of surfactant on surface tension of nanofluids is greater than nanoparticles, some researchers thought that the reduction in surface tension by surfactant SDBS can produce a superior enhancement of pool boiling performance in R141b-based nano-refrigerant [131].
Conclusions
The first part of the review focuses on the preparation and two properties viz. viscosity and surface tension of TiO2 nanofluids. It can be concluded that although one-step method is expected to achieve better dispersion stability, the side effects of the one-step method such as producing by-product and requiring special solution environment seem more fatal because they severely restrict the application scope of nanofluids. Suitable treatments such as adding dispersant, adjusting pH values, and physical means (stirring and sonication) used singly or in combination can greatly improve the dispersion stability. And the two-step method is recommended to be employed with appropriate post-treatment for the preparation of TiO2 nanofluids.
Particle loading is positively correlated to the viscosity, but the effects of other factors are not unified. The viscosities greatly differ in different researches which make the viscosity models hard to predict the experimental value, and hence, the experimental mean is firstly recommended. The surface tension of TiO2 nanofluids is more sensitive to surfactant than nanoparticles. The surfactant with low concentration is suggested to be used when it not brings obvious increase in viscosity and foaming ability due to the potential advantages such as reduction in surface tension and improvement in re-dispersible property.
Nanomaterialien
- Eigenschaften und Verwendungen von Wolframflussmitteln
- Eigenschaften und Anwendungen von Tantal
- Eigenschaften und Verbindungen von Rhenium
- Herstellung und magnetische Eigenschaften von kobaltdotierten FeMn2O4-Spinell-Nanopartikeln
- Auf dem Weg zu TiO2-Nanofluiden – Teil 2:Anwendungen und Herausforderungen
- Herstellung und photokatalytische Leistung von Hohlstruktur-LiNb3O8-Photokatalysatoren
- Strukturelle und im sichtbaren Infrarotbereich sichtbare optische Eigenschaften von Cr-dotiertem TiO2 für farbige kühle Pigmente
- Magnetische Poly(N-isopropylacrylamid)-Nanokomposite:Einfluss des Herstellungsverfahrens auf die antibakteriellen Eigenschaften
- Eigenschaften und Verwendungszweck:Aluminiumbronze
- Eigenschaften und Zusammensetzung von Roheisen