Klassifizierung, Synthese und Anwendung von lumineszierenden Silica-Nanopartikeln:eine Übersicht
Zusammenfassung
Lumineszierende Materialien sind wegen ihrer einzigartigen optischen Eigenschaften von weltweitem Interesse. Die lichtdurchlässige Kieselsäure ist eine ideale Matrix für Leuchtstoffe. Lumineszierende Siliziumdioxid-Nanopartikel (LSNs) haben aufgrund ihrer verbesserten chemischen und thermischen Stabilität breite Anwendungen. Silica-Kugeln verschiedener Größen könnten durch verschiedene Verfahren synthetisiert werden, um spezifische Anforderungen zu erfüllen. Diverse lumineszierende Farbstoffe haben Potenzial für unterschiedliche Anwendungen. In Abhängigkeit von vielen Faktoren wie Quenchern war ihre Leistung nicht ganz zufriedenstellend. Dieser Aufsatz diskutiert daher die Entwicklung von LSNs einschließlich ihrer Klassifizierung, Synthese und Anwendung. Es ist das Highlight, wie Silica die Eigenschaften von Lumineszenzfarbstoffen verbessert und welche Rolle Silica im System spielt. Außerdem werden ihre Anwendungen in Biologie, Display und Sensoren beschrieben.
Einführung
Lumineszierende Materialien sind aufgrund ihrer besonderen optischen Eigenschaften weit verbreitet [1]. Ihre Anwendung ist jedoch durch viele Einschränkungen, wie geringe Hydrophobie und Biokompatibilität, oder aufgrund von Nachteilen, wie hohe Toxizität, schlechte Biokompatibilität und geringe Absorption, eingeschränkt [2,3,4,5]. Daher ist es notwendig, lumineszierende Materialien zu modifizieren, um die Anforderungen praktischer Anwendungen zu erfüllen.
LSNs mit verbesserten Eigenschaften finden in der Biologie [6, 7], in der Beleuchtung [8] und in der Sensorik [9] zunehmend Beachtung. Ihre charakteristischen optischen Eigenschaften machen sie einzigartig in optischen Materialien [10]. Siliziumdioxid ist lichtdurchlässig, was Siliziumdioxid zu einem idealen Kandidaten als Matrix für fluoreszierende Materialien macht. Thermodynamische und chemische Stabilität sind ebenfalls wichtige Faktoren, da eine Matrix und Kieselsäure mit diesen grundlegenden Faktoren zusammenfällt [11]. Darüber hinaus kann die Oberfläche von Kieselsäure leicht modifiziert werden, was eine weitere Funktionalisierung mit verschiedenen funktionellen Gruppen ermöglicht, um unterschiedlichen Anforderungen gerecht zu werden [12]. Kieselsäure mit vielen der oben genannten Vorteile ist natürlich ein ideales Substrat zur Verbesserung der Eigenschaften von Leuchtstoffen [13]. Multifunktionale Nanosysteme können durch Zusammenfügen, Einkapseln oder Integrieren eines oder mehrerer verschiedener Nanomaterialien in und auf der Oberfläche von Siliziumdioxid-Nanopartikeln unter Verwendung verschiedener Verfahren hergestellt werden [11]. Als modifizierende Leuchtstoffe finden LSNs mit den hervorragenden Eigenschaften in der Pionierforschung zunehmend Beachtung [14]. Montalti et al. fassten viele exzellente Forschungen in der medizinischen Bildgebung mit mit organischen Farbstoffen dotiertem Siliziumdioxid zusammen [6]. Silica bietet eine stabile und multifunktionale Plattform für Phosphore, aber die Langzeittoxizität muss untersucht werden. Michael Schäfrling demonstrierte die Kunst der Lumineszenzsensoren [9]. Selektivität und Empfindlichkeit sind das Herzstück der Sensormaterialien. Zou Hua et al. über die Möglichkeiten der Modifizierung von organischem Siliziumdioxid ausgearbeitet. Nanokomposite haben gegenüber getrennten Komponenten überlegene Eigenschaften [15]. Es gibt viele Wunderberichte, die sich auf einen bestimmten Bereich wie die Biologie konzentrieren [6, 7, 16], denen jedoch eine systematische Einführung in LSNs und ihre hervorragende Leistung in anderen Bereichen fehlt.
Diese Übersicht beginnt mit der Klassifizierung von LSNs und ihren Synthesemethoden. Die Kategorien der LSNs werden systematisch anhand der Klassifikation der Leuchtstoffe festgelegt. In Bezug auf chemische Eigenschaften und Lumineszenzmechanismen sind organische Molekülfarbstoffe, lumineszierende Metalle und Quantenpunkte (QDs) dotierte Leuchtstoffe drei typische Leuchtstoffe, die alle ihre eigenen einzigartigen Lumineszenzmechanismen und Vorteile als Vertreter von LSNs haben [17,18,19 ]. Das Highlight ist, wie die Kieselsäure die Eigenschaften von Leuchtstoffen verbessert. Angesichts des Mangels an Leuchtstoffanwendungen werden die möglichen Strategien diskutiert, um ihre Leistung für LSNs zu verbessern. Dabei geht es nicht nur um biologische Anwendungen, sondern auch um Displays und Sensoren.
Klassifikationen von LSNs
Lumineszenz, die verschiedene Helligkeiten emittiert, hat einen großen Wert in materiellen Bereichen [20]. Es wurden viele Untersuchungen zur Modifikation von Leuchtstoffen durchgeführt, um das Signal-Rausch-Verhältnis, die Stabilität und die Anpassungsfähigkeit an die Umgebung für potenzielle Anwendungen zu verbessern. Die Einführung von Antennenliganden in Lanthanoidkomplexe zur Verbesserung der Lumineszenzleistung ist ein typisches Beispiel für eine Modifikation. Kieselsäure ist eine gute Matrix zum Mischen von Materialien mit unterschiedlichen Funktionen und unterschiedlichen chemischen Eigenschaften. Leuchtstoffe wurden in die Siliziumdioxidmatrix dotiert, um ihre natürlichen Defekte zu modifizieren und ihre Eigenschaften zu verbessern, was für breite Anwendungen mit leicht modifizierten und ungiftigen Siliziumdioxidoberflächen und zum Schutz von Lumineszenzfarbstoffen von Vorteil ist. Mit Multifunktionalität und einstellbarer Anpassungsfähigkeit haben LSNs immer mehr Aufmerksamkeit auf sich gezogen. Unter allen lumineszierenden Phosphoren sind organische lumineszierende Moleküle, lumineszierende metalldotierte Phosphore und QDs die drei repräsentativsten Kategorien, die es verdienen, hervorgehoben zu werden. Daher werden die obigen drei Farbstoffe als typische LSNs in Kombination mit Silica gezeigt. Repräsentative Beispiele sind in Tabelle 1 aufgeführt.
Organische Lumineszenzmoleküle dotierte LSNs
Organische Lumineszenzmoleküle sind wichtige Lumineszenzmaterialien mit π-konjugierten Ringstrukturen und geringer Größe [16]. Allerdings erschweren unspezifische Markierungen und Bleichen ihre Anwendung. Mit organischen Farbstoffen dotierte Silica-Nanopartikel werden mit ausgezeichneter Stabilität, Selektivität und Biokompatibilität umfassend untersucht [52, 53]. Van Blaaderenet al. [21] machten einen ersten Versuch, lumineszierende Siliciumdioxidkugeln zu synthetisieren. Fluoresceinisothiocyanat (FITC) wurde mit Hilfe von APS ((3-Aminopropyl)triethoxysilan) auf die Oberfläche von Kieselsäure aufgetragen, was einen praktikablen Weg zur Kombination von Farbstoffen mit Kieselsäure durch kovalente Bindungen bot. Inspiriert von diesem Prozess haben Andrew et al. [22] synthetisierten fluoreszierende Dual-Emission-Siliciumdioxid-Nanopartikel mit zwei Schichten. Zwei Farbstoffe, Tetramethylrhodaminisothiocyanat (TRITC) und FITC, wurden mittels APS in einer wasserfreien Stickstoffumgebung an die Kieselsäure konjugiert. Das schematische Diagramm und das REM-Bild (Abb. 1a) zeigten die Nanostrukturen der Nanopartikel. Als Kern der Dual-Emissions-Nanopartikel wurde zunächst Silica mit TRITC synthetisiert und FITC mit weiterem Tetraethoxysilan (TEOS) auf der Oberfläche des Kerns konjugiert. Die synthetisierten fluoreszierenden Silica-Nanopartikel mit dualer Emission untersuchten erfolgreich den intrazellulären pH-Wert in Mastzellen der basophilen Leukämie (RBL-2H3) der Ratte in Abb. 1b–d.
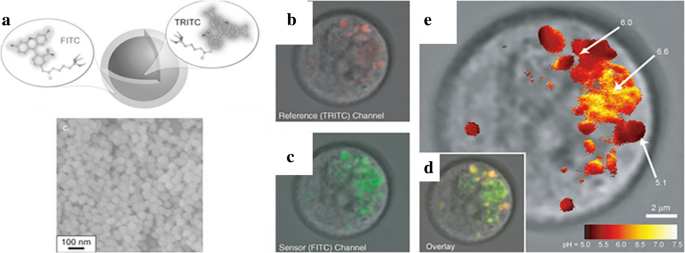
a Das Formationsdiagramm und das Rasterelektronenmikroskop (REM)-Bild von Dual-Emission-fluoreszierenden Siliziumdioxid-Nanopartikeln mit Referenzfarbstoff (TRITC) und Sensorfarbstoff (FITC); das konfokale Fluoreszenzmikroskopiebild von RBL-Mastzellen (rot als TRITC-Siliciumdioxidpartikel und grün als AlexaFluor 488-Cholera-Toxin B); konfokale fluoreszenzmikroskopische Aufnahmen von RBL-Mastzellen als pH-Sensoren. b Für den Referenzkanal c für Sensorkanal, d für die überlagerten Bilder und e ratiometrische Falschfarbenbildgebung für pH-Werte, die nach den Experimenten berechnet wurden [22]
Um die Photostabilität organischer Fluorophore zu verbessern, ist die Verkapselung von Siliciumdioxid ein häufig verwendetes Verfahren zur Modifikation. LongJiaoet al. [23] wählten vier Aminocyaninfarbstoffe als Nahinfrarot(NIR)-Fluoreszenzsonden und kuppelten sie entsprechend mit 3-Aminopropyltriethoxysilan (APTES), einem gängigen Silankupplungsmittel. TEOS hydrolysiert mit den behandelten NIR-Farbstoffen im Mikroemulsionssystem. Die mit Cyanin beladenen fluoreszierenden Silica-Nanopartikel (FSNPs) wurden nach Zentrifugation und Waschen erhalten. Der gesamte Vorgang ist in Abb. 2a dargestellt. Wie in Abb. 2b zu sehen ist, zeigten die vier in die Kieselsäure eingekapselten FSNPs eine bessere pH-Stabilität der Fluoreszenzintensität als die von freien Farbstoffen. Die vier FSNPs verbesserten gleichzeitig ihre Helligkeit (Abb. 2c). Sie testeten ihre Photostabilität in lebenden Zellen mit einem konfokalen Laser-Scanning-Mikroskop (CLSM). FSNP-3 und FSNP-4 (mehr Verankerungsstellen) erhielten eine verbesserte Photostabilität als die von freien Farbstoffen, während FSNP-1 und FSNP-2 keine Verbesserung erzielten. Mehr Verankerungsstellen verstärkten die Struktur des Farbstoffmoleküls. Ein Farbstoff mit starrer Struktur hatte einen weniger strahlungslosen Zerfall und eine harte intramolekulare Rotation, die den Farbstoff heller macht. Die Siliziumdioxidschicht kann die eingekapselten Materialien mit einer verstärkten Molekülstruktur schützen und ihre Helligkeit ohne Photobleichen verbessern. FSNP-3 und 4 hatten auch eine geringe biologische Toxizität gemäß der Methyltetrazolium-(MTT)-Methode in Abb. 2d. Biokompatibilität ist ein weiterer Vorteil von Kieselsäure.
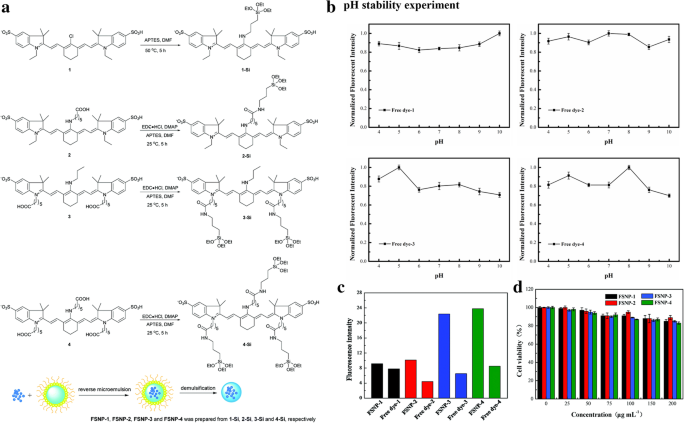
a Der Mechanismus von FSNP-1, FSNP-2, FSNP-3 und FSNP-4. b Die normalisierte Intensität bei verschiedenen pH-Werten aller Proben. c Emissionsintensität der FSNPs und freien Farbstoffe. d Zeigt nach 24 Stunden Inkubation mit FSNPs die Lebensfähigkeit der rohen 264.7-Makrophagenzellen [23]
Agglomeration ist bei den meisten Lumineszenzfarbstoffen einer der Hauptgründe für das Quenchen. Leuchtstoffe können mit Silica stetig in einer angemessenen Konzentration gehalten werden. Aggregationsinduzierte Emissionsluminogene (AIEgens) leiden im Gegensatz zu den herkömmlichen Luminophoren nicht unter diesem Problem. Umgekehrt führt die Aggregation zu einer starken Emission [54]. Um die Leistung von AIEgens in biologischen Bereichen zu verbessern, werden viele Polymermatrizen verwendet, um AIEgens einzukapseln. Darüber hinaus gibt es einige andere Probleme, die zum Quenchen von AIEgens führen können, wie z. B. Wasser und Sauerstoff, was sich negativ auf die Anwendung auswirkt. Kieselsäure kann sie von den Quenchern abhalten [55]. Basierend auf dieser obigen Analyse wurde TPETPAFN (ein typisches Fluorogen bestehend aus zwei Tetraphenylethylen-Anhängern und einem intramolekularen Ladungstransferkern), ein AIEgen, durch F127 (Poly(ethylenglycol)-Block-Poly(Propylenglycol)-Block-Poly ( Ethylenglykol)) zur Kernmicelle [24]. TEOS wurde hydrolysiert, um über ein modifiziertes Sol-Gel-Verfahren eine auf die Kernmicelle beschichtete Siliciumdioxidhülle zu erhalten. Wie in Abb. 3 gezeigt, ist das synthetische TPETPAFN-F127-SiO2 Nanopartikel wiesen bessere Photolumineszenzeigenschaften auf und profitierten vom Schutz der Siliziumdioxidhülle.
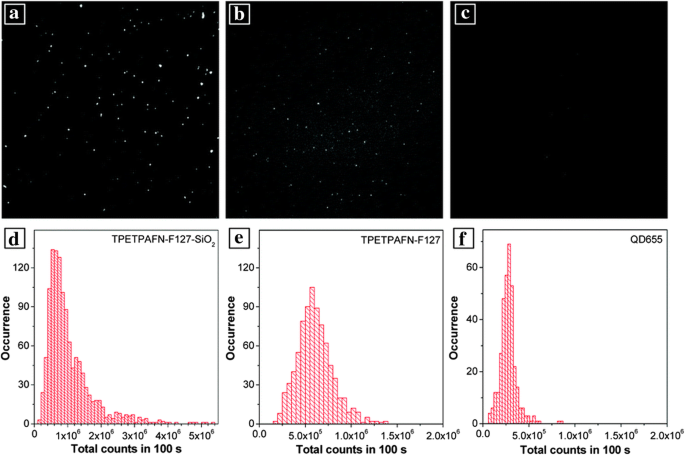
a , d Anzeige der Fluoreszenzbilder und der Histogramme der Photonen von TPETPAFN-F127-SiO2 NPs, entsprechende b , e für TPETOAFN-NPs und c , f für kommerziellen QD655 [24]
Leuchtende metalldotierte LSNs
Seltenerdmetall [56] und Übergangsmetall [57] sind gängige lumineszierende Metallmaterialien, die auf dem Ladungstransferübergang basieren. Lumineszenz nach Komplexierung mit dem Liganden ist das offensichtlichste Merkmal dieses Materials. Es gibt zwei Hauptmechanismen der Metalllumineszenz, LMCT (Ligand-Metall-Ladungsübergang) und MLCT (Metall-Ligand-Ladungsübergang). Lanthanoidmetalle und Übergangsmetalle sind jeweils ihre typischen Beispiele. Aufgrund der reichlich vorhandenen elektronischen Energieniveaus gibt es verschiedene lumineszierende Metalle, die ein großes Anwendungspotenzial im Bereich der Lumineszenz mit unterschiedlicher Emission haben [58]. Edelmetalle mit LSPR werden häufig in Materialien mit verstärkter Lumineszenz verwendet und werden in diesem Abschnitt behandelt. Dennoch schränken die geringe Sensibilisierungseffizienz und das Quenchen die Anwendungen von lumineszierenden Metallen ein [59]. Um ihre Photostabilität und Biokompatibilität zu verbessern, haben Francis et al. fügte den Liganden für die weiteren Modifikationen eine substituierte Silylgruppe hinzu [30]. Eu@Si-OH-Nanopartikel wurden erhalten, nachdem silylgruppenmodifizierte Eu-Komplexe mit Siliciumdioxid über ein Umkehrmikroemulsionsverfahren beschichtet wurden. Das Produkt wurde schließlich mit APTES als Eu@Si-NH2 . aminfunktionalisiert Nanopartikel. Die Kieselsäureschicht hielt die Komplexe von den Quenchern (OH und NH2 Gruppen). Als Ergebnis zeigten beide eine bessere Photostabilität in Abb. 4. Eu@Si-NH2 Nanopartikel zeigten gute Leistungen im Bioimaging.
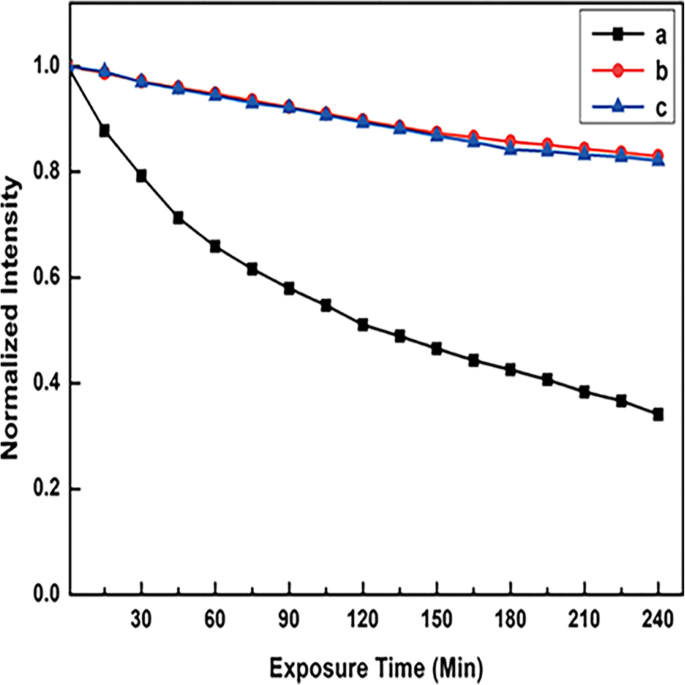
Die Kurven der Fluoreszenzintensität, die sich mit der Belichtungszeit unter 365 nm-Bestrahlung ändern, a Eltern-Eu-Komplex in CHCl3 Lösung, b Eu@Si-NH2 , und c Eu@Si-OH-Nanopartikel in phosphatgepufferter Salzlösung (PBS) [30]
Ezquerroet al. integrierte Ir-Komplexe, MLCT-Lumineszenzkomplex, in das Siliciumdioxidgerüst für verbesserte Stabilität und photophysikalische Eigenschaften über einen Sol-Gel-Prozess [60]. Mit dem Schutz von Siliziumdioxid zeigten diese Leuchtstoffe eine ausgezeichnete Stabilität nicht nur unter Umgebungsbedingungen, sondern auch unter rauen Umgebungen, die weitere Anwendung in weißen Leuchtdioden (WLED) finden.
Y.Liet al. [31] synthetisierten ölsäurestabilisierte Upconversion-Nanopartikel (UCNPs). Dann beschichteten sie die UCNPs über ein Mikroemulsionsverfahren mit einer Siliziumdioxidschicht, und es wurden wasserlösliche UCNPs erhalten. Einführung in Eu (TTA)3 Phen-Komplexe in das System, synthetisierten sie NaGdF4 :Yb,Er@SiO2 @Eu (TTA)3 Phen (UCNPs@SiO2 @EuTP) Nanokugeln. Die Oberflächenabschreckung wurde nach der Silikabeschichtung unterdrückt und als Ergebnis erhöhte sich die Emissionsintensität, wie in Abb. 5 gezeigt. Die wasserlöslichen Nanopartikel mit zwei unterschiedlichen Emissionen wurden mit Hilfe von Silica erhalten.
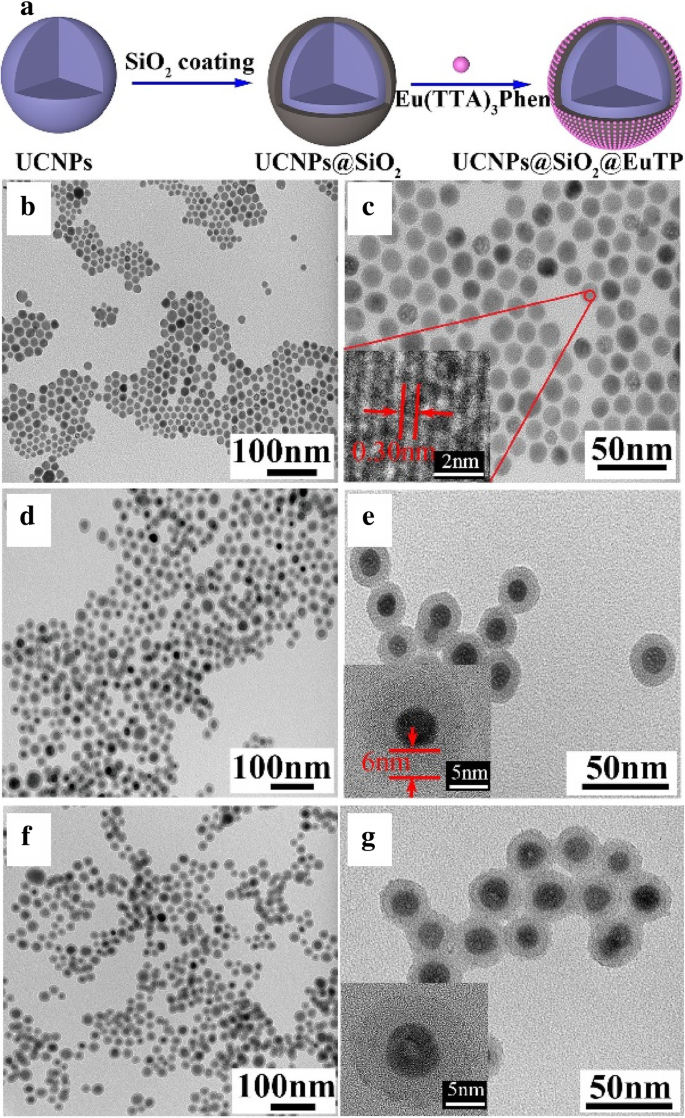
a Die Bildung von UCNPs@SiO2 @EuTP und TEM (Transmissionselektronenmikroskop) Bilder der Proben; b , c für UCNPs, d , e für UCNPs@SiO2 , und f , g für UCNPs@SiO2 @EuTP [31]
Chenet al. [42] setzten erfolgreich Kohlenstoffpunkte (CDs) und Seltenerd-Ionen für WLED ein. Sie synthetisierten CDs mit der organischen Eintopf-Pyrolysemethode, die ein Maximum der blauen Emission bei 470 nm und zwei Anregungspeaks bei 251 bzw. 364 nm aufwiesen. Um ein weißes Licht emittierendes Komposit zu erhalten, wurden CDs als blauer Emissionskern und Sr2 . verwendet Si5 N8 :Eu 2+ Phosphor wurde als orangefarbene Emissionskomponente verwendet. CDs wurden in das Stöber-System eingelegt. Wenn TEOS hydrolysiert wird, werden CDs mit einer Siliziumdioxidschicht mit rotem Phosphor beschichtet. Der Kohlenstoff-Punkt-Silica-Phosphor-Verbundstoff (CDSP) wurde nach Zentrifugieren, Trocknen und Mahlen synthetisiert. CDSP hatte eine breite Absorption vom UV (Ultraviolett) bis zum gelben Bereich (200–600 nm), besonders stark im UV-Bereich. Nachdem sie die Anregung bei verschiedenen Wellenlängen getestet hatten, stellten sie fest, dass das CDSP bei der Anregung bei 400 nm in Abb. 6. Und es war ein guter Versuch, die Emission von CDSP durch Abstimmung des Massenverhältnisses von CD und Phosphor zu erhalten. Unter Anregung bei 400 nm erreichten sie das nächste Massenverhältnis (3,9 % (0,32; 0,32) und 5,1 % (0,34; 0,32)) zur weißen Emission. CDSP zeigte eine bessere weiße Emission (0,30, 0,31) in Leuchtdioden-(LED)-Verpackungen als die von CD&P (direkte Vermischung mit CDs und Phosphor) (0,28, 0,29). Zwei Komponenten dispergierten homogen mit Siliciumdioxid und verringerten die Wahrscheinlichkeit von Aggregation und Phasentrennung. Schließlich erhielten sie eine WLED mit CDSP-Pulver auf einem UV-Diodenchip (375 nm) und erhielten weißes Licht (0,30, 0,33). Der Farbwiedergabeindex (CRI) war etwa 94, höher als der der kommerziellen WLED auf YAG:Ce-Basis (CRI < 75).
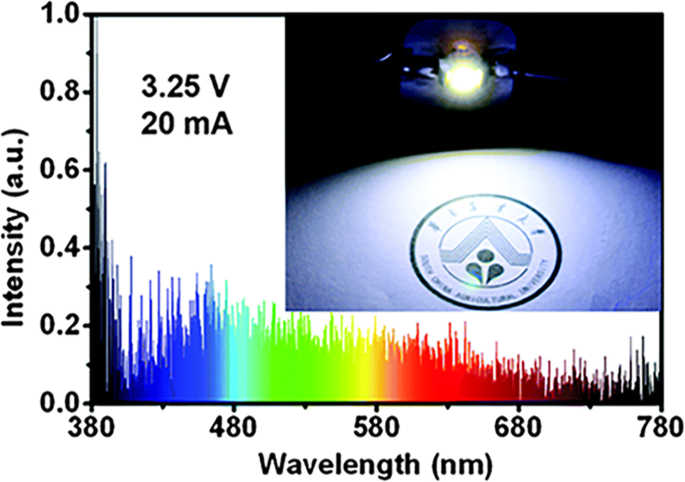
Die Leistungen von CDSP-basierter WLED:Emissionsspektren und Fotografie [42]
Siliziumdioxid wird üblicherweise als Schutzschicht für Leuchtstoffe verwendet, um den richtigen Abstand zu Edelmetallen zu halten, um die Fluoreszenz zu verstärken. Dies ist auf die stehende Schwingung freier Elektronen zurückzuführen, die durch Licht verursacht wird. Um die Lumineszenz zu verstärken, muss ein entsprechender Abstand zwischen Farbstoffen und Edelmetallpartikeln eingehalten werden. Bei edel verstärkten Materialien können Metallnanopartikel selbst das Löschen der Chromophore verursachen (innerhalb von 5 nm), aber ihre Fluoreszenz kann sich bis auf das 100-fache erhöhen (bei etwa 10 nm). In der frühen Forschung haben Tuo Li et al. [61] synthetisierten Ag-Nanopartikel mit der Siliciumdioxidhülle in der Mikroemulsionsmatrix (Ag/SiO2 Nanopartikel). Die zur Herstellung von Siliciumdioxid benötigten Reagenzien (TEOS und Cyclohexan) wurden nach der Silberreduzierung in die Mikroemulsion injiziert. Sie untersuchten sorgfältig die Auswirkungen verschiedener Bedingungen (Wasser/Tensid für R und Wasser/TEOS für H) auf das Ag/SiO2 . Nanopartikel und die Ergebnisse sind in Abb. 7 dargestellt. Es ist ein guter Weg, nicht nur Ag, sondern auch andere Nanopartikel mit Mikroemulsionssystem auf den Kern mit einer gleichmäßigen und dicken Siliziumdioxidschicht zu beschichten. Was Zhenhua Bai et al. [25] tat ist ein gutes Beispiel. 8-Hydroxypyren-1,3,6-tresulfonsäure (HPTS), eine Art fluoreszierender pH-empfindlicher Farbstoffe, eignet sich aufgrund seiner einzigartigen Vorteile für die Herstellung als intrazezelluläre pH-Sensoren. Aber extreme pH-Bedingungen machten es unempfindlich. Wenn die Lösung sauer ist, würde ihre Fluoreszenzeffizienz erheblich abnehmen. HPTS-adsorbiertes Ag@SiO2 Nanopartikel (Abb. 8a) wurden basierend auf einem durch Edelmetalle verstärkten Fluoreszenzeffekt hergestellt. Aus Abb. 8b ist ersichtlich, dass Ag@SiO2 -8 nm@HPTS zeigte insbesondere bei extremen pH-Bedingungen eine bessere Fluoreszenzintensität.
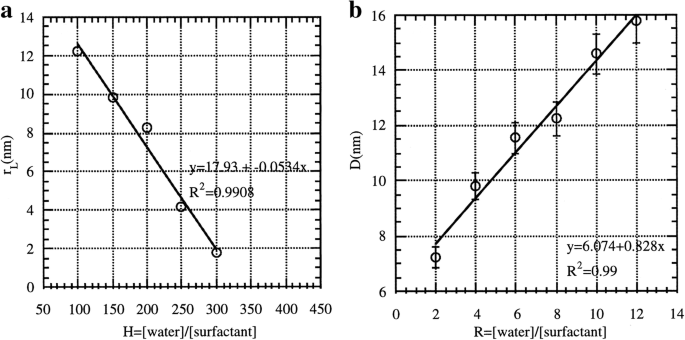
a Die Größenänderung als Funktion von H (R = 4 und X = 1); b wenn R ist variabel, die Größenänderung von Ag-Clustern [61]
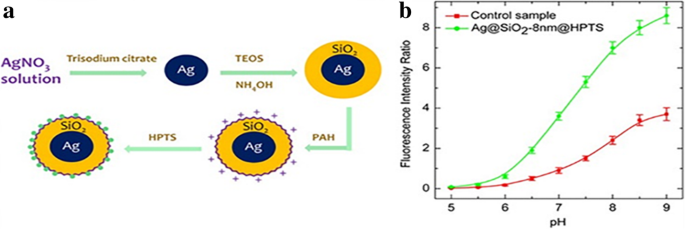
a Der Fortschritt der Synthese von HPTS-adsorbiertem Ag@SiO2 Nanopartikel. b Das Fluoreszenzintensitätsverhältnis von Ag@SiO2 -8 nm@HPTS (grün) und Kontrollprobe (rot) [25]
QDs-dotierte LSNs
Aufgrund des Quanteneinschlusseffekts weisen QDs ausgezeichnete Lumineszenzeigenschaften auf, unabhängig davon, ob es sich um Halbleiter-QDs, Kohlenstoff-QDs oder andere Typen handelt. In letzter Zeit haben sich zahlreiche Studien auf die Anwendungen von QDs in optischen Geräten konzentriert. Manchmal reichen ihre Eigenschaften nicht aus, um die komplexen Anwendungen anzupassen. Notwendige Modifikationen sind zwingend erforderlich und Kieselsäure ist eine geeignete Matrix [1].
Um die Kombination von Biolabel und Magnetresonanztomographie zu realisieren, wurden CdSe-QDs auf das magnetische Fe2 . aufgetragen O3 Kern durch eine Silikatschicht mit NH2 Gruppe. Die zugehörigen Bilder und Charakterisierungen sind in Abb. 9 gezeigt. Kombiniertes NH2 Gruppe mit bioverankerter Membran (BAM), 4 T1 Brustkrebszellmembranen von Mäusen zeigten eine spezifische Markierung mit BAM-SiO2 -CdSe-MQDs [44]. Mit der Biokompatibilität und Magnetkraft würden die multifunktionalen Lumineszenz-Nanopartikel breite Anwendung in der Medizin finden.
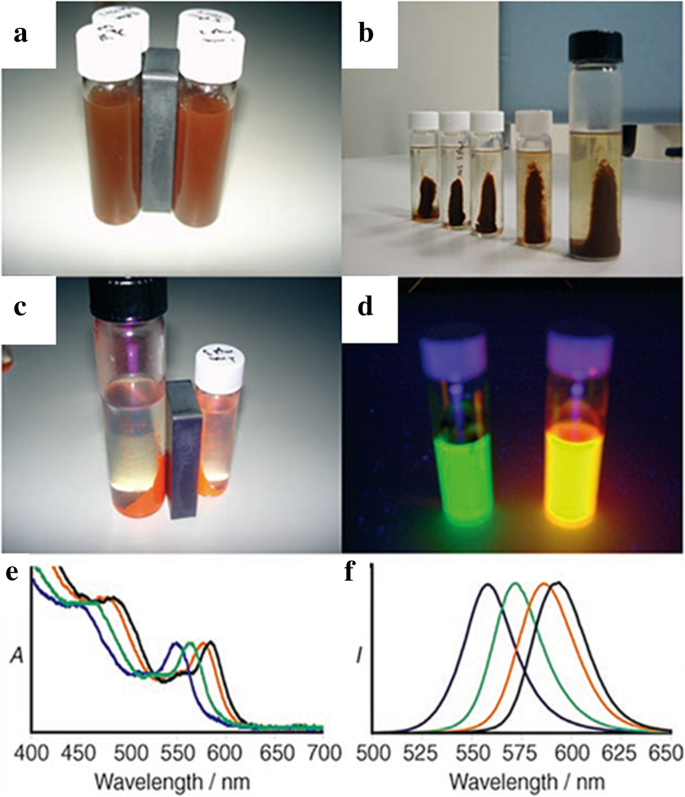
Die Fotos bei normalem Licht zum Nachweis der magnetischen Modifikation (a , b ). c Die Fotografie unter UV-Licht, um sowohl die magnetischen als auch die Lumineszenzeigenschaften zu beweisen. d Die UV-Fotografie grüner und oranger magnetischer Quantenpunkte (MQDs). e , f Die Lumineszenzspektren von SiO2 -MQDs in PBS-Lösungen (e zur Absorption und f für Emission) [44]
Um die Anwendung von QDs zu erweitern, ist es notwendig, ihre Wasserlöslichkeit und Ungiftigkeit zu modifizieren. Silica zeigt großes Potenzial bei der Modifikation von QDs. Yunfei Ma et al. [43] führten ein selbst hergestelltes Phasentransferreagenz (Adenosin 5′-monophosphat, AMP) und ein Silankupplungsmittel (3-Mercaptopropyltrimethoxysilan, MPS) in das Stöber-System ein. Öllöslich (anfängliche CdSe/CdS/ZnS-QDs), alkohollöslich (AMP-QDs) und wasserlöslich (Hydrolyse von TEOS um die QDs herum) waren der gesamte Fortschritt der Löslichkeitsänderung. QD@SiO2 hatte die gleiche Photolumineszenz-Effizienz (50–65%) wie die ursprüngliche. Ein breiterer pH-Bereich (pH 4–8 bis 2–13), verbesserte Stabilität im Elektrolyten, bessere thermische Stabilität und erhöhte Biokompatibilität in Hela-Zellen waren die Vorteile des QD@SiO2 .
Um die Stabilität von QDs in optischen Geräten zu gewährleisten, ist es notwendig, den Effekt des Blinkens zu reduzieren. Blinken ist ein Phänomen mit einer zufälligen intermittierenden Lumineszenz, das die Stabilität der optischen QDs-Geräte beeinflusst [62]. Um die Wirkung des Blinzelns zu reduzieren, haben Botao Ji et al. [45] stellten CdSe/CdS-QDs als Kernmaterialien her und kapselten diese QDs basierend auf der Verdrängung der anfänglichen hydrophoben Liganden durch eine Umkehrmikroemulsionsmethode in eine Siliciumdioxidhülle ein. Und die QDs weiter modifiziert durch eine Au-Schicht auf der Oberfläche von Siliciumdioxid mit Poly(1-vinylimidazol-co-vinyltrimethoxysilan) (PVIS) als Silankupplungsmittel. Die Goldhülle in Nanogröße fungierte als Plasmonenresonator, der den QDs eine verbesserte optische Zustandsdichte verlieh. Die Eigenschaften der QDs können aufgrund der Hybridschicht unabhängig von drastischen Änderungen in der lokalen Umgebung erhalten bleiben. Dadurch erhöhte sich die Photostabilität der QDs. Die QD-Fluoreszenzlebensdauer reduzierte sich nach der Goldbeschichtung von 123 auf 20 ns. Die goldenen QDs zeigten eine effiziente Multiexzitonenemission und ihre neutrale Photolumineszenzintensität war höher als die von QDs. Die Ergebnisse des Stabilitätstests sind in Abb. 10 dargestellt. Außerdem konnte die Photolumineszenzintensität über mehrere Stunden (sogar 24 h) stabil bleiben. Der Lumineszenzstabilitätstest zeigte, dass die Lumineszenz von bloßen QDs nach nur 1 h einen dramatischen Abfall aufweisen würde. Die Siliziumdioxidschicht verbesserte die Leistung von QDs geringfügig, bot jedoch das geeignete Intervall für die nächste Au-Schicht, um den plasmaverstärkten Effekt zu zeigen.
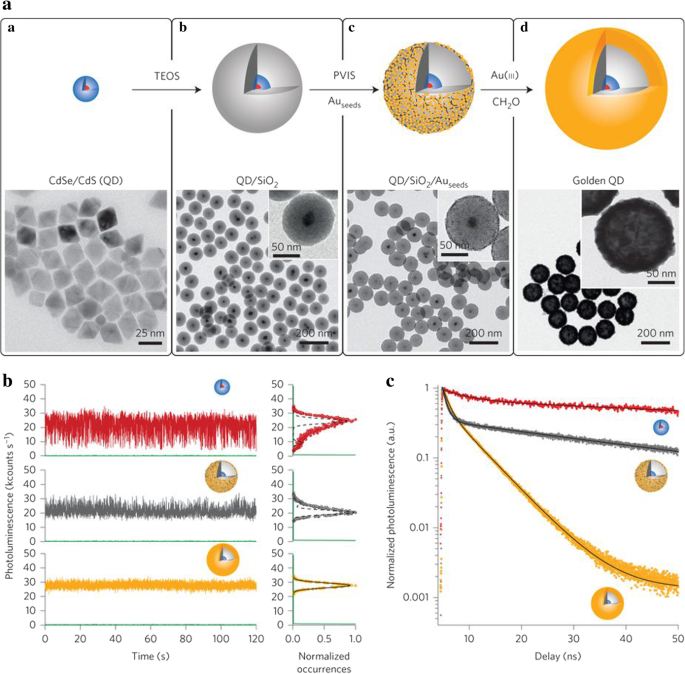
a Schema Quantenpunkt/SiO2 /Au-Hybrid (goldenes QD) und die TEM-Bilder der einzelnen Stufen (CdSe/CdS-QDs, QD/SiO2 QD/SiO2 /AuSamen und goldene QDs). b Die zeitliche Änderung der Photolumineszenzintensität. Rot für CdSe/CdS, grau für QD/SiO2 /AuSamen , und orange für goldene QDs. c Photolumineszenz-Zerfallskurven von drei Nanopartikeln in (b ) [45]
Synthetische Methoden von LSNs
Bei der Herstellung von LSNs sind die Auswahl der Leuchtstoffe und das Design von Synthesewegen die Kerninhalte. Phosphore bestimmen den Emissionsbereich von LSNs und Syntheserouten legen ihre Strukturen und Funktionen fest. Alle Synthesewege von LSNs basieren auf der Kieselsäure. Das Sol-Gel-Verfahren, das Umkehrmikroemulsionsverfahren und das direkte Micellen-Hilfsverfahren sind drei Hauptansätze, um homogene und regelmäßige Siliciumdioxidkugeln zu erhalten, die in LSNs verwendet wurden. Abbildung 11 ist das schematische Diagramm der genannten Methoden.
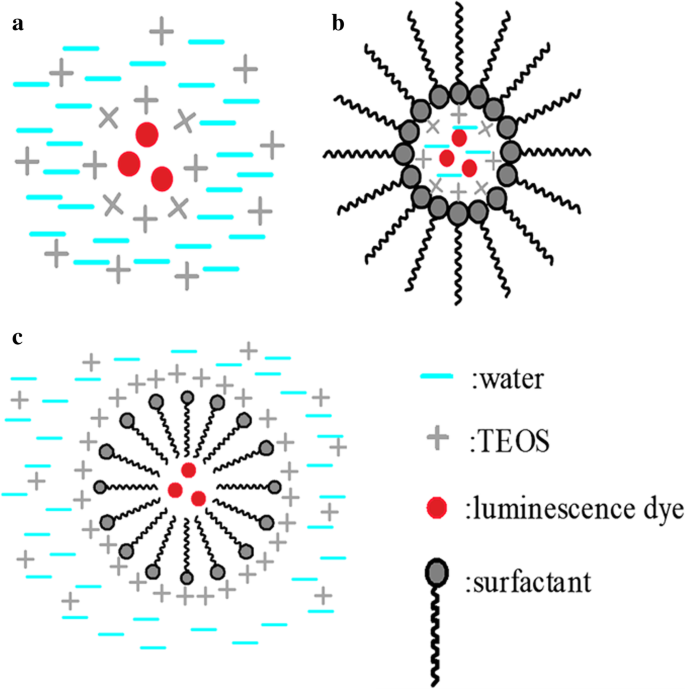
Die schematischen Darstellungen verschiedener LSNs mit unterschiedlichen Methoden. a Für das Stöber-Verfahren. b Für die umgekehrte Mikroemulsionsmethode, c Für direkte Micellen-Assistentenmethode
Sol-Gel-Methode
Das Sol-Gel-Verfahren, auch Stöber-Verfahren genannt, ist ein bequemes und durchführbares Verfahren, um monodisperse Siliziumdioxid-Nanokügelchen zu erhalten. Es ist ideal, Silica-Nanokugeln zu synthetisieren, da Stöber [63] die Synthese von größenspezifischen Silica-Kugeln im Bereich von 50 nm–2 μm mit Alkoxysilanhydrolyse unter Ammoniakkatalyse sorgfältig untersucht hat. Homogene Silica-Kugeln mit unterschiedlichen Größen (10 bis einige Hundert Nanometer) können leicht durch Kontrolle der Synthesebedingungen wie des Ethanol-zu-Wasser-Verhältnisses, der Ammoniakmenge und der Temperatur über das Sol-Gel-Verfahren erhalten werden. Mit der Stöber-Methode synthetisierten Van Blaaderen und A. Vrij Langmuir erfolgreich mit Farbstoff (FITC) dotiertes Siliciumdioxid durch Zugabe von (APS) in das Reaktionssystem [21]. Mit der Amingruppe von APS fingen Silica-Kugeln FITC leicht ein, wie in Abb. 11a gezeigt. Bisher wurden neben Farbstoffen viele andere Materialien über die Stöber-Methode mit Kieselsäure verknüpft. Luis M. Liz-Marzan et al. verbesserten die Stöber-Methode und synthetisierten Gold-Silica-Kern-Schale-Partikel unter Verwendung von (3-Aminopropyl)-trimethoxysilan (APTS) als Tensid [64]. In Kombination mit dem Goldkern bietet APTES eine chemische Bindungsüberbrückung für die Siliziumdioxid-Einkapselung. Alkalische Bedingungen führen zu homogenen Silica-Kugeln als beliebtes Stöber-System, und die säurekatalysierte Hydrolyse von Alkoxysilan ist auch eine praktikable Methode, um Lumineszenzfarbstoffe in Silica einzukapseln [65].
Basierend auf der Stöber-Methode wurde eine neue Art von LSNs synthetisiert. Lingang Yanget al. [50] synthetisierten erfolgreich kristallines Siliciumdioxid nach der Stöber-Methode, basierend auf der π-π-Stapelung von Vinylgruppen. Ein Stöber-Fortschritt mit Vinyltriethoxysilan (VTES) als Vorstufe, Neutralisation mit Salzsäure, Vakuumdestillation zur Entfernung des Lösungsmittels und Extraktion mit Tetrahydrofuran sind die ganzen Verfahren der Organosilica-Nanokristalle (OSNCs). Drei OSNCs wurden mit derselben Kristallstruktur, aber in unterschiedlichen Größen synthetisiert, wie in Abb. 12d–f gezeigt. Die Größe von Organosilica-Nanokristallen (OSNCs) nimmt aufgrund der Zunahme von VTES allmählich zu. Als Ergebnis zeigten sie unterschiedliche Lumineszenzeigenschaften, wie für Abb. 12g, h (blau, grün und rot unter UV-Licht) gezeigt. OSNCs wurden durch gute Photostabilität und pH-Stabilität charakterisiert. Das epitaktische Wachstum von Vinylgruppen in einer kubischen Diamantkristallstruktur wird aufgrund der π-π-Stapelung dargestellt. Die geordneten gestapelten Vinylgruppen bilden schließlich ein großes π-konjugiertes System mit Fluoreszenz nach dem Quanteneinschluss. Diese OSNCs hatten aufgrund der Eigenschaften von Siliziumdioxid ein großes Potenzial auf dem optischen Gebiet, was einen neuen Ansatz zur Herstellung von selbstlumineszenten Siliziumdioxidmaterialien darstellte.
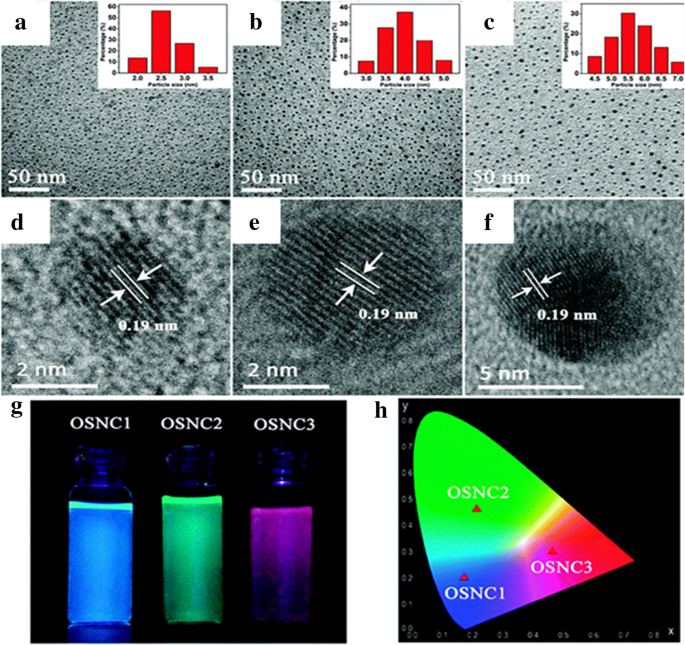
Charakterisierungen von OSNCs:a –c als TEM-Bilder und d –f als hochauflösende Transmissionselektronenmikroskopie (HRTEM) Bilder. g Die Fotografien von OSNC-Proben unter UV-Licht-Beleuchtung. h Die Verteilung auf der Farbtafel der Commission Internationale de l’Eclairage (CIE) [50]
Umgekehrte Mikroemulsionsmethode
Die Stöber-Methode ist eine einfache und bequeme Methode zur Synthese von LSNs, aber Reaktionsbedingungen und Ausgangspartikel, die nicht kontrolliert werden, schränken die Lumineszenzfarbstoffe ein. Um solche Einschränkungen zu überwinden, führten Bagwe und Khilar [66] das Wasser-in-Öl-Mikroemulsionssystem [67] während der Synthese von mit Silica-Nanokompositen beschichtetem Silber ein (Abb. 11b). Die anfängliche alkalische wässrige Lösung von Silbernanopartikeln mit TEOS wurde mit Tensiden in den Wassertropfen eingekapselt. Der Hydrolyseverlauf von TEOS war der gleiche wie der des Stöber-Verfahrens. Aber der ganze Fortschritt beschränkte sich auf Wassertröpfchen, die von Tensiden eingeschlossen waren, was zu einem gut kontrollierten System und monodispersen Siliciumdioxid-Nanopartikeln führte. Die Größe des Siliciumdioxids wurde durch Auswahl verschiedener Tenside, Lösungsmittel und Änderung des Verhältnisses von Tensid zu Wasser gut kontrolliert. Wenn die Fluorophore wasserlöslich sind, bildet sich innerhalb der Moleküle im Tröpfchen leicht eine homogene Siliziumdioxidschicht auf der Oberfläche. Nianfang Wang et al. [46] synthetisierten lumineszierende, silikabeschichtete CdS/CdSe/CdS-Nanopartikel über eine Umkehrmikroemulsionsmethode. Abbildung 13 zeigt die TEM-Bilder der synthetischen QDs und QDs@SiO2 . Die geschützten QDs zeigten eine ausgezeichnete Säure- und Wärmestabilität. Es bot die Möglichkeit für weitere Modifikationen, um spezielle Anforderungen für Anwendungen zu erfüllen.
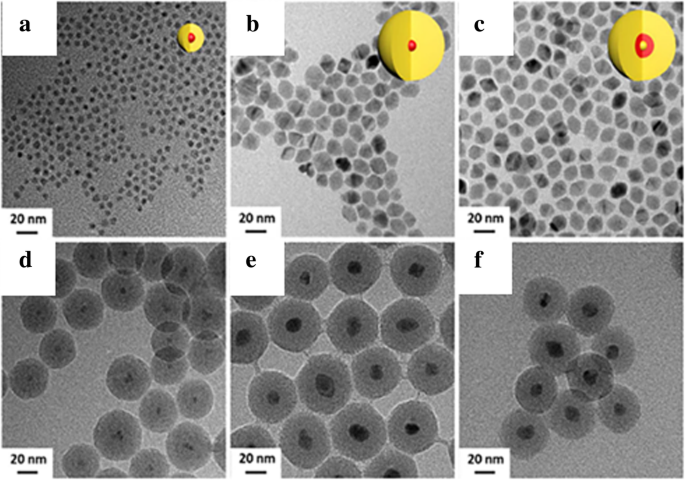
TEM-Bilder von CdSe/CdS-Kern/Schale-QDs mit dünner CdS-Schale (a ) und nach Beschichtung mit Silica CdSe/CdS@SiO2 (d ); CdSe/CdS-Kern/Schale-QDs mit CdS-Schale (b ) und nach Beschichtung mit Silica CdSe/CdS@SiO2 (e ); CdS/CdSe/CdS core/shell QDs (c ) and after coating with silica CdS/CdSe/CdS@SiO2 (f ) [46]
Direct Micelles Assistant Method
Reverse microemulsion method require the water-soluble luminescence dyes. Inversely, liposoluble initial micelles are the major features of direct micelle method, and the hydrolysis progress takes place around of the micelles (Fig. 11c). A precursor is indispensable for the agglomeration of silica. As a common progress, the luminescent dye is modified with the silane coupling agent, such as APS, to form the assistant micelles. The initial modified micelles ensure that the TEOS condensation occurs around them. Using Rhodamine B conjugated to APTES as the original micelle, Kumar et al. [26] successfully synthesized Rhodamine-conjugated organically modified silica nanoparticles in oil in water system and modified them with different function groups (such as sulfhydryl, amino, and carbonyl) which can be used as cell fluorescence probe.
The role of the surfactant is not only reflected in the silica synthesis but also in the synthesis of mesoporous silica. A common method of synthesizing mesoporous silica is calcination. Large specific surface area and modifiable surfaces make the mesoporous silica nanoparticles perfect carriers. In addition to the known application value in the field of medical drug loading, it also has important application prospects in the field of loading phosphors. Li Wang et al. [68] mixed up CDs with hollow mesoporous silica microspheres with good photochemical stability which can be used for oxygen detection in the whole range. Mesoporous structure makes them unique. Bin Xie et al. [69] incorporated the CdSe/ZnS core-shell QDs into mesoporous silica microspheres by a swelling and evaporation method. Coated with a mesoporous silica layer on the surface of Gd2 O3 :Eu phosphors via modified Stöber method is also feasible according to the Ali Aldalbahi et al. [70]. Because of the encapsulation of silica, the modified Gd2 O3 :Eu nanoparticles showed excellent solubility and biocompatibility.
Other Methods
There are also other methods to synthesize LSNs such as chemical vapor deposition (CVD) [71], hydrothermal method [51], and amino acid-catalyzed seed regrowth technique [72, 73].
Lianzhen Cao et al. [71] synthesized SiC/SiO2 by CVD and thermal annealing processes. Si was used to coat on the SiC core by thermal CVD and then SiO2 shell was obtained after oxidizing. The annealed SiC/SiO2 nanoparticles showed narrow luminescence in the blue-green region. The synthetic method provided a new way to synthesize core-shell nanomaterials.
Chandra et al. [51] synthesized smaller fluorescent silica nanoparticles (1 to 2 nm) with silicon tetrabromide (SiBr4 ) and APTS. Heating to 200 °C in an autoclave was the core step of the whole reaction. The final products were obtained after further purification including dialysis and centrifugation. The silica nanoparticles emitted bright blue luminescence with a photoluminescence quantum yield around 34%. It was non-photobleaching and biocompatible at the same time.
Surface modification makes the LSNs more tunable for complex application [74]. Silane coupling agents are the most common chemical methods as it mentions before. Abundant hydroxyl groups provide reaction sites for further modifications. Junqiang Wang et al. synthesized silica modified CeO2 ammonia sensor with high gas response due to hydroxyl groups [75]. After hydrolysis and condensation, silane coupling agents with different function groups bond on the surface of silica. Superhydrophobic silica was synthesized with the condensation of VTES (-CH=CH2 ) [76]. Ming Ma et al. grafted PEGMA and DMEAA on the surface by RAFT polymerization based on the -NH2 of APTS [77]. Surface modification can enhance their adaptability in complex environments and get improved luminescence properties with appropriate materials.
Among these methods, there are two main ideas to fabricate LSNs, namely the luminescent dyes are added directly into the reaction system when the silica resources start hydrolyzing, and that the luminescent dyes are established chemical bond with silica by other reagents such as silane coupling agents, either before or after silica network set up. It is necessary to select and design an appropriate synthetic route for LSNs with specific structures.
Applications of Luminescent Silica Nanoparticles
Light is the most intuitive tool for people to recognize the world. Luminescent materials with special emission can be directly used in many ways such as lighting, display, and so on. At the same time, changes in fluorescence intensity can reflect some important information. Compared with separate luminescent dyes, LSNs have improved performances in applications, since silica provides a stable matrix for the luminescent dye. It provides an effective way for multifunction at the same time [6]. LSNs with multifunction and tunable surface have great application prospects and development potential in biology, lighting, and sensors.
Biolabeling and Medicine
LSNs have great application value in biology. Non-toxicity is a fundamental requirement for medical field, especially in vivo [78]. The fact that the common luminescent dyes are often toxic limits their clinical application [79]. Silica, a favorite non-toxic modified material, is a good solution to elimination of toxicity. Toxicity of silica nanoparticles (20–200 nm) were also carefully studied by In-Yong Kim et al. [80]. Size, dose, and cell type-dependent cytotoxicity were the issues in their research. Although high dose can cause a disproportionate decrease in cell viability, the silica nanospheres with 60 nm showed their good biocompatibility up to 10 μg/ml. Different cells had different tolerance to silica nanoparticles which indicated that it was necessary to have substantial tests before clinical tests. Although inhalation of silica particles can cause acute and chronic diseases including silicosis [81], silica still has potential in biological application at the nanoscale. The toxicity of luminescent silica nanoparticles to living cells was studied in detail by Yuhui Jin et al. [38]. From the DNA level to the cell level, the toxicity of RuBpy-doped LSNs were carefully tested. At a certain concentration, the results showed that the dye-doped luminescent silica nanoparticles were non-toxic to the targeted DNA and cells, which indicate that LSNs are a good solution to the non-toxic modification. Xiqi Zhang et al. [27] encapsulated AIE dye (An18, derivatized from 9, 10-distyrylanthracene with an alkoxyl endgroup) into the silica nanoparticles via a one-pot modified Stöber method. Coated with silica lead to an enhanced fluorescence intensity, good water solubility, and non-toxicity to living cells which made the An18-SiO2 NPs had a potential for biomedical application.
LSNs have great application value in diagnosis and biolabeling. For hybrid imaging contrast agents, Dong Kee Yi et al. [48] combined magnetic particles (MPs) Fe2 O3 with QDs (CdSe) and encapsulated them in silica shell by reverse microemulsion method. The nanostructures of MPs with QDs are clearly showed in Fig. 14. Magnetic resonance imaging (MRI) is an effective method for disease detection, especially for cancer. The advantages of feasible usage, low cost, and accurate diagnosis make it more popular as a diagnostic tool [7]. The nanocomposites can be used as both optical and MRI contrast agents. It is worth mentioning that the presence of CdSe increased the effective magnetic anisotropy of the γ-Fe2 O3 -containg particles. This is a good attempt, but the low quantum yield (SiO2 /MP-QD 1.1% to CdSe 11.4%) limits the actual effect. Willam J. Rieter et al. [39] also synthesized the same multifunctional nanocomposites. What is different is that [Ru (bpy)3 ] Cl2 was chosen as the luminescent core and the paramagnetic Gd complex was coated on the luminescent core by water-in-oil reverse microemulsion method. The nanocomposites were finally embedded in silica in the same way. The results of Fig. 15 proved that hybrid silica nanoparticles had good optical and MRI performances in biological imaging. Mesoporous silica nanospheres doped with europium (Eu-MSN) were obtained by Mengchao Shi et al. [32]. Nanoscale size (280–300 nm) and fluorescent property were the basic for an ideal biolabeling material. They found that Eu-MSNs had a positive influence on osteogenesis and angiogenesis-induction. By promoting proper response of macrophages and the expression of relevant genes, the defect of bone replaced by new bone and the healing process of skin wound can accelerate with Eu-MSNs. Besides the function of biolabeling, the LSNs showed the potential in tissue repair. LSNs can achieve the target binding effect by modifying the special group. In Duarte’s work [33], organosilane Bpy-Si was chosen as a ligand of Eu complex for the further reaction with silica. SiO2 -[Eu (TTA)3 (Bpy-Si)] nanoparticles were obtained with a uniform size (28 ± 2 nm). With a further modification of an amino acid spacer and an anchor group (anti-Escherichia coli , IgG1), the functionalized silica had the specific bonding with E . coli Bakterien. It was easy to get the distribution of E . coli bacteria with luminescence. The bio-multifunction of LSNs was also carefully studied by Laranjeira et al. [82]. Gadolinium (Gd) composites with unique magnetic properties have potential in MRI contrast agents but Gd3+ ions are toxic in humans especially in kidneys and pancreas. GdOHCO3 nanoparticles were chosen as the MRI contrast core and coated with silica layer via Stöber method. With the silica coating, the Gd composite (SiGdOHCO3 ) had the same brightness of MRI contrast images but no degradation at designed pH values (5.5, 6.0, and 7.4). And SiGdOHCO3 had little effect on human fibroblasts according to the cell proliferation assay which indicated an excellent biocompatibility. Silica provides a more stable environment and further possible modification for GdOHCO3 without affecting MRI performance. By diverse micelles method, Atabaev et al. [83] synthesized Gd2 O3 :Tb 3+ ,Eu 3+ @SiO2 nanoparticles which can be used as both MRI contrast and fluorescence agents in vivo. The above two examples perfectly reflected the role of LSNs in multifunction with the silica platform.
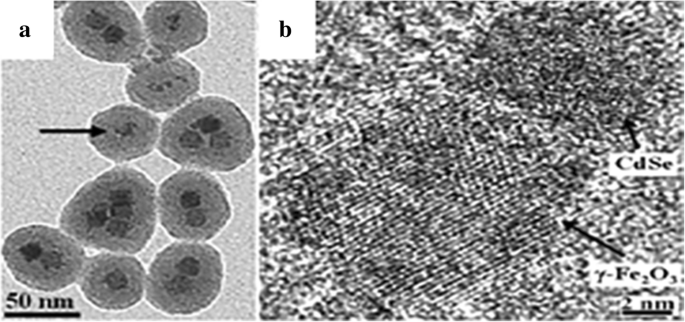
a TEM image and b HRTEM image of SiO2 /MP-QD nanoparticles [48]
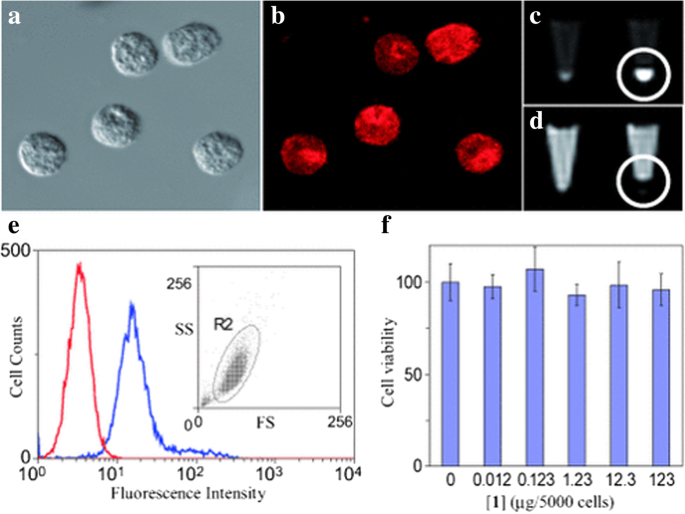
The imaging results of monocyte cells with a optical microscopic, b laser scanning confocal fluorescence, c , d the images of monocyte cells with MR:left for unlabeled monocyte cells and right for hybrid silica nanoparticles labeled monocyte cells, e flow cytometric results of blank and hybrid silica nanoparticles-labeled monocyte cells, and f the cell viability with different amount of hybrid silica nanoparticles [39]
LSNs have great application value in drug delivery. Hongmin Chen synthesized luminescent mesoporous silica nanoparticles biofunctionalized by targeting motifs, which makes them applicable in drug delivery [47]. They first prepared APS-containing mesoporous silica particles, and subjected the products to calcination at 400 for 2 h. They synthesized mesoporous silica by the help of cetyltrimethyl ammonium bromide (CTAB). There were luminescent carbon dots in the silica matrix after calcination. The fluorescence intensity was at the maximum when the particles were excited at 380 nm. The target selectivity of FL-SiO2 was achieved by surface modification of RGD peptide with the help of APS. They also studied the RGD-FL-SiO2 loading and release of doxorubicin (Dox). After calcination, fluorescent mesoporous silica (FL-SiO2 ) can still load Dox effectively. The porous structure was not affected by calcination. They found that RGD-FL-SiO2 had good luminescent effect especially around the blood vessels of tumor in vivo imaging studies. Integrin αv β3 of the tumor was the key of the interactions. Although there are many excellent attempts to apply LSNs to medicine but less successful clinical tests in human beings means that there is still a long way to go for the real medicine applications.
Light-Emitting Devices
Due to their special emitting features, LSNs also play a vital role in light emission fields including the field emission- and liquid crystal-based display technologies [84]. WLEDs have received recent attention for their broad applications including general illumination and displays. Tunable color, high color purity, and luminescence efficiency are in line with the requirements of light-emitting diodes (LEDs) [85]. Quantum dot-based light-emitting diodes (QD-LEDs) have demonstrated recently, and may offer many advantages over conventional LED and organic light-emitting diodes (OLEDs) technologies in terms of color purity, stability, and production cost, while still achieving similar levels of efficiency. In order to improve the performances of polymer dots (Pdots) as WLED phosphors, Kaiwen Chang et al. [49] introduced some Pdots with different emission wavelength into the Stöber system to get encapsulated. The silica-encapsulated Pdots showed the same luminescence properties but markedly enhanced photostability.
To reduce the manufacturing complexity required for achieving full-color displays, it is more desirable to use a common device structure to achieve high efficiency for three primary colors (blue, green, and red). QDs have been widely used in display field because of its unique luminescent properties, such as high luminescent intensity, narrow emission spectra, and tunable emission. Chun Sun et al. [34] synthesized the perovskite QDs, CsPbBr3 , as the light-emitting core of WLEDS. Only the perovskite QDs are not enough for a LED device since photostability and stability are necessary for an optical device under long-time work and elevated temperature. There are anion-exchange reactions between different halide QD nanoparticles which would widen the narrow emission spectrum. QD/silica composite were fabricated in APS to avoid oxidation and decomposition. So they used APTES as the QDs’ capping agent and improved the silica coating process to avoid the decomposition of the QDs. Green and red QD/silica composites were synthesized and a WLED was obtained by the combination of the composites with a blue LED chip. The WLED had good performances with great air stability as depicted in Fig. 16.
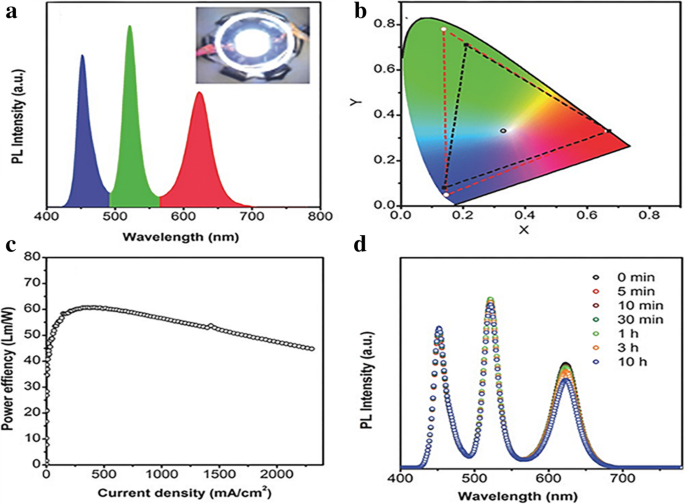
The optical performances of the WLED:a the emission spectra, b the CIE color coordinates and the color triangle of WLED (red dashed line) with the NTSC TV standard (black dashed line), c the power efficiency, and d emission spectra after working for a while [34]
LSNs can keep good dispersion, brightness, and photostability of QDs. Hung-Chia Wang et al. [35] provided a new composite method for QDs and silica (Fig. 17). By mixing the QDs with mesoporous silica powder of which pore size was bigger than that of QDs in non-polar solution, mesoporous silica green PQD nanocomposite was obtained after washing and drying. The quantum dot showed better thermal stability and photostability after composited with silica. On the other hand, QDs are a typical kind of aggregation-caused quenching (ACQ) nanoparticles, which means that it is necessary to keep a good dispersion to get a good brightness and photostability. Kai Jiang et al. [86] synthesized carbon dots with red, green, and blue luminescence with phenylenediamines as precursors to enhance luminescence properties as solution and poly (vinylalcohol) (PVA) film. But it would exist quenching effect as solid-state CDs which was fatal for LED devices owing to aggregation and the result Förster resonance energy transfer (FRET). To avoid the dispersion and the resulting FRET phenomenon, Junli Wang et al. [36] embedded carbon dots into silica matrix (Fig. 18) by dispersing carbon dots into the N -(3-(trimethoxysilyl)propyl) ethylenediamine (KH-792) and heating to form a homogenous CD/silica film. A white LED was fabricated by drying the CD/silica solution on the inner wall. By the assistant of silica, CDs were well dispersed with an appropriate distance without quenching which improve the performance as powders. Figure 18 showed the emission spectra and performance in WLED. And the CIE coordinates (0.44, 0.42) and correlated color temperature (CCT) (2951 K) suggested that it was suitable for indoor illumination.
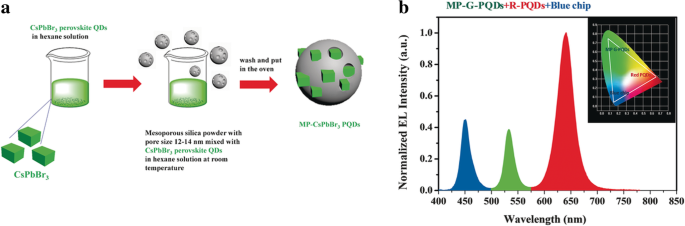
a The formation progress of MP-CsPbBr3PQDs. b The luminescence intensity and the color triangle of WLED [35]
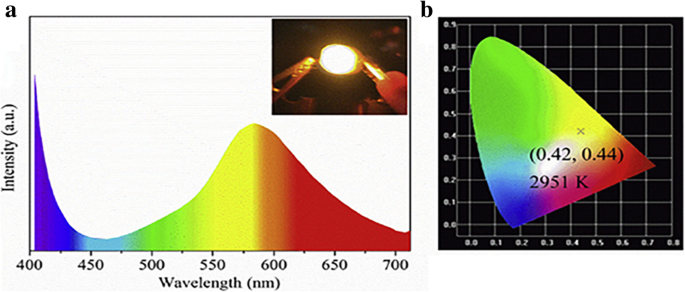
The performance of WLED showed as a the emission spectrum and b for CIE chromaticity and CCT [36]
Sensors
Luminescent silica showed the excellent performances on static luminescent materials, such as biolabeling and WLED phosphors. All these were based on their unique and stable optical properties. When it came to dynamic luminescent materials, LSNs also display the same wonder [9]. The luminescent sensors of pH [28], ions [87], and temperature [40] are following as representatives.
pH value have great influence on the luminescence intensity which inspires luminescent pH sensor. In the same principle as ref. [22], Atabaev et al. synthesized the same ratiometric pH sensor [28]. FITC was chosen as the pH-dependent luminescence dye and Y2 O3 :Eu 3+ as pH stable dye. With the Stöber coating of silica, Y2 O3 :Eu 3+ @SiO2 with FITC composite NPs were successfully synthesized. The change of pH was reflected by the ratio of fluorescence intensity (I FITC /Ich Y2O3:Eu3+ ). The standard dye led to a less influence of concentration and a more accurate result.
LSNs can also be used as ions sensors. Based on the changes of luminescence intensity with the measured physical quantity, LSNs have been applied to many sensor fields by the environment-dependent effect of the luminescence. Quenching effect is an effective detective tool to detect the changes of quenching factors such as ions and pH value with external quenching mechanisms such as FRET and photoinduced electron-transfer (PET) [9]. Sensors for metal ions are important fields whether in cells or open system. Won Cho et al. [37] synthesized europium (III) coordination polymer (EuCP) and found the specific quenching effect of Cu 2+ (Fig. 19). In view of this fact, they synthesized silica@EuCP microsphere which have the same sensitivity on Cu 2+ with less mass of europium. As an auxiliary material, silica can effectively reduce the amount of sensor materials. Both of them have their unique situations. Besides quenching effect, there are some different effects which can be used in the fields of sensors. 2,2-Dipicolylamine (DPA) and its derivatives have good affinity to heavy ions. And enhanced luminescence effect would happen after chelated with heavy ions. Yu Ding et al. [29] modified silica spheres with N ,N ′-bis (pyridine-2-ylmethyl)ethane-1,2-diamine (Fig. 20). The concentration of heavy ions (Cd 2+ Hg 2+ and Pb 2+ ) in samples can be determined by the change of fluorescence intensity. The test in real water samples and simulated biological samples confirmed the heavy metal ions-binding ability and the detection which has application prospects in the water monitoring and so on.
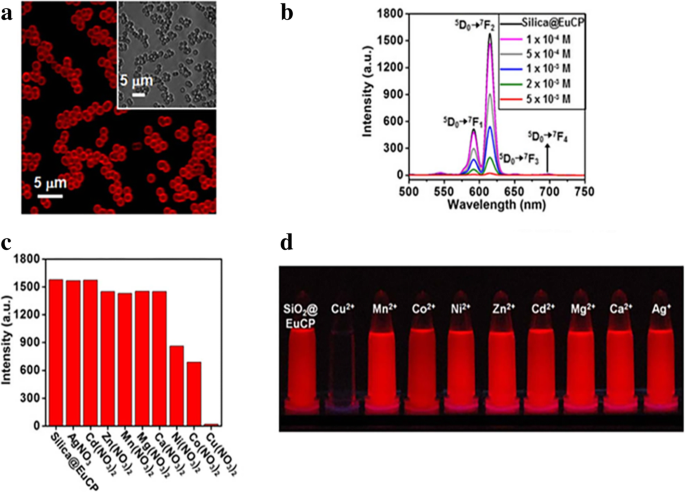
a Confocal microscopy and OM (inset) images of silica@EuCP microspheres. b Luminescence spectra with different Cu (NO3 )2 in MeCN; luminescence intensity changes (c ) and photograph (d ) with different metal ion solutions (5 mM) [37]
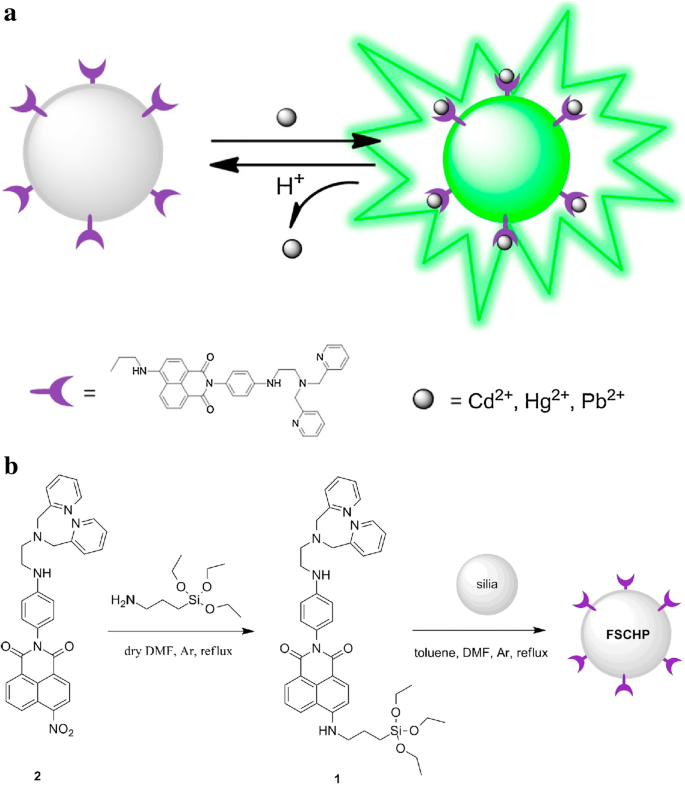
The formation and sensing progress scheme of sensitive fluorescent sensor (FSCHP) [29]
Temperature sensors are also important applications of LSNs. Temperature is a basic variable in most science fields. The temperature dependence of radiative and non-radiative transition rates is the core content of temperature sensing which makes it possible for luminescence temperature sensing, with the contactless and large-scale advantages [9]. However, in order to be applied in practice, their stability is crucial as the environment of application is more complex than of that of experiment condition. Silica is an ideal matrix to improve their performance for application. Mirenda et al. [40] synthesized silica as the core and then TEOS was hydrolyzed with Ru (bpy)3 Cl2 to form the Ru (bpy)3 @SiO2 NPs. The emission spectra of Ru (bpy)3 @SiO2 NPs (Fig. 21) showed that the intensity of Ru (bpy)3 @SiO2 NPs decreased linearly as the temperature rising as the result of the activated non-radiative 3 d-d state (20–60 °C, λexc = 463 nm). The polyethyleneimine (PEI)-modified glass with Ru (bpy)3 @SiO2 NPs showed the same trend as the NPs which proved that the potential as the temperature sensing. With cycling the temperature between 20 and 60 °C, the relative slope decreased until the seventh cycle which meant that it is necessary to condition to obtain the stable sensing materials. The influence of temperature on probes is complicated. So it is necessary to research the temperature-dependent luminescence of the probes to know how to apply it into temperature sensors. Temperature is a fundamental variable that governs diverse intracellular chemical and physical interactions in the life cycle of biological cells. The change of temperature reflects the level of cell metabolism. GdVO4 co-doped with Er 3+ (1 mol%) and Yb 3+ (1 mol%) has the potential to apply as the temperature sensor. To improve their performance as temperature sensor, Savchuk et al. [41] coated silica shell on the nanoparticles surface by Stöber method. The fluorescence intensity ratio (FIR) of Er, Yb:GdVO4 , I 520 /Ich 550 , had a certain linear relationship with temperature in the range from 297 to 343 K after excitation at 980 nm. And the probes got enhanced thermal sensitivity, high thermal resolution and good stability in different solvents. And the result of the ex vivo experiment to monitor temperature evolution with the special sensor showed in Fig. 22 proved that Er, Yb:GdVO4 @SiO2 core-shell nanoparticles had a good thermal resolution as the temperature sensor in biomedical applications.
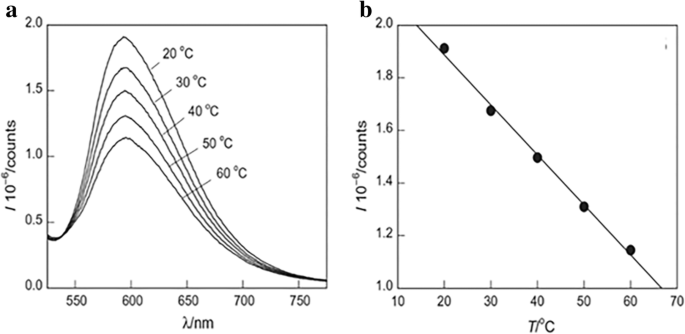
a PL spectra of Ru (bpy)3 @SiO2 under different temperature. b The peak intensity changes as a function of temperature [40]
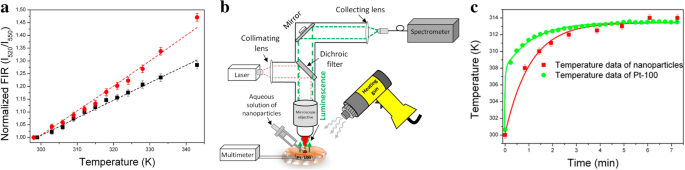
a I 520 /Ich 550 with different temperature for Er, Yb:GdVO4 and Er, Yb:GdVO4 @SiO2 . b The sketch map for the ex vivo temperature determination experiment. c The results of the temporal evolution of temperature for the Er, Yb:GdVO4 @SiO2 and a thermoresistor Pt-100 [41]
Conclusion
In this article, LSNs with various functions demonstrate that silica is an ideal host material for luminescent dyes. The visualization of related parameters is the most special feature of luminescent dyes. Various luminescent materials have their own advantages but there are still some defects which limit their applications. Improved brightness, photostability, and thermal stability are the advantages of LSNs with the protection of silica. At the same time, it provides phosphors with a versatile platform which makes it possible to become multifunctional and specially modified. Excellent performance, adjustable adaptability, and potential versatility broaden the applications of fluorescent materials. LSNs have great potential in many unmentioned fields such as solar cells and photocatalysts. However, there is still a long way to apply LSNs to the actual species. Poor selectivity and low signal-to-noise ratio in complex conditions are factors that constrain LSNs for the practical applications which need to be further studied. Defined distances between phosphors and LSPR metal deserve more investigations to get the positive effect. Many new luminescent materials with excellent luminescence properties have been developed which means that it is necessary to improve the traditional synthetic methods to obtain LSNs. Silica is a traditional modified material but LSNs still have great potential for development.
Abkürzungen
- ACQ:
-
Aggregation-caused quenching
- AIEgen:
-
Aggregation-induced emission luminogens
- AMP:
-
Adenosine 5′-monophosphate
- An18:
-
An aggregation-induced emission-based organic fluorogen derivatized from 9,10-distyrylanthracene with alkoxyl endgroup
- APS:
-
(3-Aminopropyl)triethoxysilan
- APTES:
-
3-Aminopropyltriethoxysilane
- APTS:
-
(3-Aminopropyl)trimethoxysilane
- B:
-
Blue
- BAM:
-
Bio-anchored membrane
- CCT:
-
Corresponding correlated color temperature
- CDs:
-
Carbon dots
- CDSP:
-
Carbon dot-silica- phosphor composite
- CIE:
-
Commission Internationale de l’Eclairage
- CLSM:
-
Confocal laser scanning microscope
- CRI:
-
Color rendering index
- CTAB:
-
Cetyltrimethyl ammonium bromide
- CVD:
-
Chemical vapor deposition
- DDT:
-
1-Dodecanethiol
- Dox:
-
Doxorubicin
- DPA:
-
2,2-Dipicolylamine
- F127:
-
Poly (ethylene glycol)-block-poly (propylene glycol)-block-poly (ethylene glycol)
- FIR:
-
Fluorescence intensity ratio
- FITC:
-
Fluorescein isothiocyanate
- FL-SiO2 :
-
Fluorescent mesoporous silica
- FRET:
-
Förster resonance energy transfer
- FSCHP:
-
Sensitive fluorescent sensor
- FSNP:
-
Fluorescent silica nanoparticle
- G:
-
Green
- H:
-
The ratio of water/TEOS
- HPTS:
-
8-Hydroxypyrene-1,3,6-tresulfonic acid
- HRTEM:
-
High resolution transmission electron microscopy
- IgG1:
-
Anti-Escherichia coli
- KH-792:
-
N-(3-(trimethoxysiyl)propyl)ethylenediamine
- LEDs:
-
Ligh-emitting diodes
- LSN:
-
Luminescent silica nanoparticle
- LSPR:
-
Local surface plasmon resonance
- MPS:
-
3-Mercaptopropyltrimethoxysilane
- MPs:
-
Magnetic particles
- MQDs:
-
Magnetic quantum dots
- MRI:
-
Magnetic resonance imaging
- MTT:
-
Methyl tetrazolium
- NIR:
-
Near-infrared
- NTSC:
-
National Television System Committee
- OLEDs:
-
Organic light-emitting diodes
- OSNC:
-
Organosilica nanocrystal
- OTES:
-
n-Octyltriethoxysilane
- PBS:
-
Phosphate-buffered saline
- Pdots:
-
Polymer dots
- PEI:
-
Polyethyleneimine
- PET:
-
Photoinduced electron transfer
- PVA:
-
Poly (vinylalcohol)
- PVIS:
-
Poly (1-vinylimidazole-co-vinyltrimethoxysilane)
- QD655:
-
A kind of commercial quantum dots
- QD-LEDs:
-
Quantum dot-based light-emitting diodes
- QDs:
-
Quantum dots
- R:
-
Red
- R:
-
The ratio of water/surfactant
- RBL-2H3:
-
Rat basophilic leukemia mast cells
- SEM:
-
Rasterelektronenmikroskop
- TEM:
-
Transmissionselektronenmikroskop
- TEOS:
-
Tetraethoxysilane
- TPETPAFN:
-
A typical fluorogen consisting of two tetraphenylethylene pendants and an intramolecular charge transfer core
- TRITC:
-
Tetramethylrhodamine isothiocyanate
- UC:
-
Upconversion
- UCNP:
-
Upconversion nanoparticles
- UCNPs@SiO2@EuTP:
-
NaGdF4:Yb,Er@SiO2@Eu (TTA)3Phen
- UV:
-
Ultraviolet
- VTES:
-
Vinyltriethoxysilane
- WLED:
-
White light-emitting diode
Nanomaterialien
- Anwendung von Wolfram und Wolframlegierungen
- Klassifizierung von Farbstoffen nach Anwendung
- Klassifizierung und Anwendung von Glasfasern
- Multifunktionale Goldnanopartikel für verbesserte diagnostische und therapeutische Anwendungen:Eine Übersicht
- Herstellung von ICA-beladenen mPEG-ICA-Nanopartikeln und ihre Anwendung bei der Behandlung von LPS-induzierter H9c2-Zellschädigung
- Biogene Synthese, Charakterisierung und Bewertung des antibakteriellen Potenzials von Kupferoxid-Nanopartikeln gegen Escherichia coli
- Eine Übersicht über die Anwendung von Biosensoren und Nanosensoren in Agrarökosystemen
- Einfache Synthese und optische Eigenschaften kleiner Selen-Nanokristalle und -Nanostäbe
- Nanostrukturiertes Siliciumdioxid/Gold-Cellulose-gebundenes Amino-POSS-Hybrid-Komposit über den Sol-Gel-Prozess und seine Eigenschaften
- Einfache Synthese von farbigen und leitfähigen CuSCN-Kompositen, die mit CuS-Nanopartikeln beschichtet sind