Ein neuartiges Design eines intelligenten Drug Delivery Systems basierend auf Nanoantennenpartikeln
Zusammenfassung
Das Wirkstoffabgabesystem aus zusammengesetzten Nanopartikeln spielt eine wichtige Rolle bei der Interaktion mit Lymphknoten. Es gibt drei Haupttypen von Lymphozyten:B-Zellen, T-Zellen und natürliche Killerzellen. Wenn die Zellen des Immunsystems krebserregend werden, greifen sie Körperzellen an. Die Lymphflüssigkeit spielt eine wichtige Rolle beim Angriff auf gesunde Zellen des Körpers; Daher zielte diese Veröffentlichung darauf ab, ein Wirkstoffabgabesystem zu entwickeln, das Nanopartikel effizient auf die infizierten Zellen lenken kann und so zur schnellen Eliminierung solcher Zellen beiträgt. Das vorgeschlagene Design hängt von der Interaktion zwischen diesen Molekülen ab, und der intelligente Nanocontroller hat die Fähigkeit, die Nanopartikel durch anaeroben Kontakt zu führen. Das vorgeschlagene Design bewies, dass die dynamische Viskosität der Flüssigkeit umso geringer ist, je kleiner die Nanopartikelgröße und -dichte ist, was ihren Strömungswiderstand widerspiegeln würde. Darüber hinaus wurde der Schluss gezogen, dass Wasserstoffmoleküle aufgrund ihrer geringen Dichte eine bedeutende Rolle bei der Verringerung des Lymphflüssigkeitswiderstands spielen.
Einführung
Die aktuellen Behandlungsmöglichkeiten von Krebs umfassen Operation, Bestrahlung und Chemotherapie. Diese Behandlungsstrategien schädigen auch normales Gewebe und führen zu einer teilweisen Vernichtung des malignen Wachstums. Daher kann die Nanotechnologie diese Mängel überwinden, indem sie gezielt schädliche Zellen und Neoplasmen angreift, Tumore direkt reseziert und die Wirksamkeit strahlenbasierter und anderer Behandlungsmodalitäten erhöht. Dies kann die Nebenwirkungen der Behandlung deutlich verringern und die Überlebensrate erhöhen. Die Nanotechnologie ist ein vielversprechendes Werkzeug für die Behandlung von bösartigem Wachstum, da sie durch den Einsatz von Nanomaterialien neue und bessere Behandlungsmethoden bietet. Nanopartikel können spezifisch auf viele Moleküle abzielen, die unterschiedlich auf Krebszellen exprimiert werden. Die im Allgemeinen große Tragflächenregion von Nanopartikeln kann mit Liganden wie kleinen Partikeln und Desoxyribonukleinsäure-korrosiven oder Ribonukleinsäure-Korrosionsketten-Peptid-Antikörpern funktionalisiert werden. Die Liganden werden als Arzneimittel und in theranostischen Anwendungen verwendet. Die physikalischen Eigenschaften von Nanopartikeln, wie z. B. Vitalitätsdistraktion und Rückstrahlung, können ebenfalls genutzt werden, um krankes Gewebe zu beeinflussen, beispielsweise bei Laserentfernungs- und Hyperthermieanwendungen [1].
Das innovative Nanopartikel-Softwareprogramm und das aktive pharmazeutische Element werden auch die Erforschung eines breiteren Repertoires an Wirkstoffen ermöglichen. Daher werden die immunogene Ladung und die Oberflächenbeschichtung sowohl als Adjuvantien zur Nanopartikel-vermittelten als auch zur traditionellen Chemotherapie untersucht. Diese innovative Strategie umfasst das Design von Nanopartikeln als künstliches Antigen, das auf Zellen und in vivo-Depots von stimulierenden Faktoren präsentiert wird, die Antitumorwirkungen ausüben. Die Nanotechnologie stellt ein aktives Forschungsgebiet mit vielen Anwendungen dar. Nanopartikel haben aufgrund ihrer einstellbaren physikalisch-chemischen Eigenschaften wie Auftauindex, Hydrophilie, elektrische und thermische Leitfähigkeit, katalytische Aktivität, Lichtabsorption und Streuung in der Medizintechnik Interesse geweckt [2]. Im Prinzip werden Nanomaterialien als Materialien mit Partikeln im Bereich von 1 bis 100 nm beschrieben. In der Europäischen Union und den USA gibt es mehrere Gesetze, die sich speziell auf die medizinische Forschung mit Nanomaterialien beziehen. Es gibt jedoch keine international anerkannte Definition von Nanomaterialien. Verschiedene Organisationen betrachten unterschiedliche Konzepte von Nanomaterialien [3]. Eines der Ziele des Nanopartikel-Wirkstoffabgabesystems ist die Behandlung von Lymphflüssigkeit mit Krebszellen. Ein System zur Wirkstoffabgabe aus zusammengesetzten Nanopartikeln in Wechselwirkung mit Lymphknoten ist in Abb. 1 dargestellt.
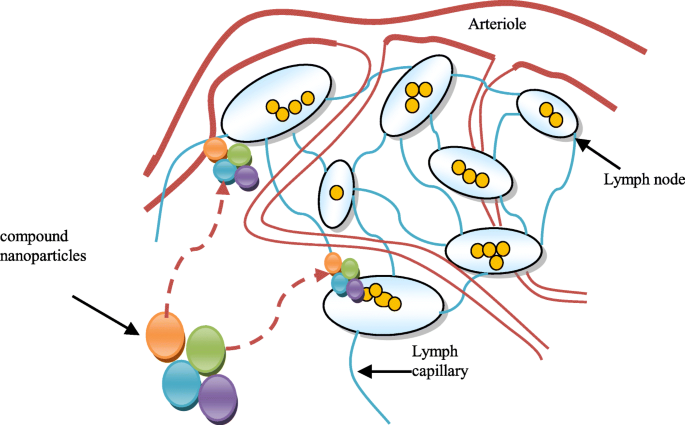
Wirkstoffabgabesystem aus zusammengesetzten Nanopartikeln und seine Wechselwirkung mit Lymphknoten
Die US-amerikanische Food and Drug Administration bezeichnet Nanomaterialien als Materialien mit Partikeln im Bereich von 1 bis 100 mit anderen Eigenschaften als das Schüttgut [4, 5]. Nanofasern, Nanoplättchen, Nanodrähte, Quantenpunkte und andere verwandte Materialien wurden charakterisiert [6]. Feste Lipid-Nanopartikel (SLNs) sind eine Art von Lipid-Nanopartikeln (LN), die unter Verwendung fester Lipide konstruiert werden können [7]. Spätere Versionen von SLNs wurden entwickelt, wie zum Beispiel nanostrukturierte Lipidträger (NLCs), die die zweite Ära der LN darstellen [8]. Sowohl SLN als auch NLC werden aus festen Lipiden aufgebaut. Die innere Struktur von SLN enthält feste Lipide, während NLC unter Verwendung einer Mischung aus festen und flüssigen Lipiden entwickelt wird, die einen Edelsteinquerschnitt erzeugen [9, 10]. Diese Mängel wurden auch für SLNs berichtet, da SLNs, die viele feste Lipidsegmente enthalten, in medizinischen Anwendungen verwendet werden können [11, 12]. Polymere Nanopartikel (PN) können aus natürlichen Polymeren oder anorganischen Materialien, beispielsweise Siliziumdioxid, aufgebaut werden [13]. Die Polymere oder Lipide formen den Kern von NPs, die die Stabilität und Wirkstoffabgabe verbessern und eine einheitliche Form und Größe bieten [14]. PN kann als Nanokapseln oder Nanokugeln beschrieben werden. Die Nanokapseln enthalten Öl in vesikulärer Struktur zusammen mit einem Wirkstoff [15, 16], während Nanokügelchen Polymerketten ohne Öl enthalten [17, 18]. Ein Medikament wird durch Mischen mit dem Polymer in PNs verpackt. Der Einbau des Wirkstoffs in die Nanopartikel wird zum Zeitpunkt der Polymerisation sichergestellt. PNs werden mit einem Wirkstoff beladen, indem dieser in den Bestandteilen des Polymernetzwerks gelöst, gestreut oder künstlich adsorbiert wird [19, 20]. Es gibt drei Arten von Lymphozyten:B-Zellen, T-Zellen und natürliche Killerzellen. Die B-Zellen produzieren Antikörper, die eindringende Mikroorganismen angreifen, während sie auch das Immunsystem angreifen, wenn sie krebserregend werden. Unter Berücksichtigung der wichtigen Rolle der Lymphflüssigkeit bei der Autoimmunität war es daher das Ziel dieser Arbeit, ein intelligentes Drug Delivery System basierend auf Nanoantennenpartikeln zu entwickeln. Somit enthält das System viele Nanopartikel in unterschiedlichen Mengen. Der nächste Abschnitt stellt das Design eines intelligenten Systems zur Verabreichung von Medikamenten vor.
Design eines nanointelligenten Drug Delivery Systems
Das vorgeschlagene nanointelligente Wirkstoffabgabesystem enthält einen Nanocontroller, der von einer elektrischen Quelle von Nanopartikeln aus einem nanopiezoelektrischen Material betrieben wird. Das komplexe Depot von Nanopartikeln verfügt über eine Reihe von Mikro-Repositorien. Jedes kleine Depot enthält eine Art von Nanopartikeln. Ein Nanopartikel-Molekül enthält eine Nanoantenne, die dafür ausgelegt ist, mit dem Nano-Controller zu kommunizieren. Das vorgeschlagene nanointelligente Wirkstoffabgabesystem enthält auch Kohlenstoff-Nanoröhrchen für die schnelle Abgabe von Wirkstoffen an Krebszellen. Es kann mit den infizierten Zellen in Verbindung gebracht werden, wie in Abb. 2 gezeigt. Das System beginnt mit dem Senden von Nanopartikeln an die Krebszellen, die als „explorative Nanopartikel“ bezeichnet werden. Diese Moleküle senden mittels anaerober Kommunikation das vollständige Bild ihrer Position innerhalb der Zellen an den Nanocontroller. Basierend auf der Situation der explorativen Nanopartikel sendet der Nanocontroller Nanopartikel unterschiedlicher Anzahl, Art und Dichte an die Krebszellen, basierend auf den von den explorativen Nanopartikeln gesammelten Informationen. Diese Nanopartikel werden „Kampf-Nanopartikel“ genannt.
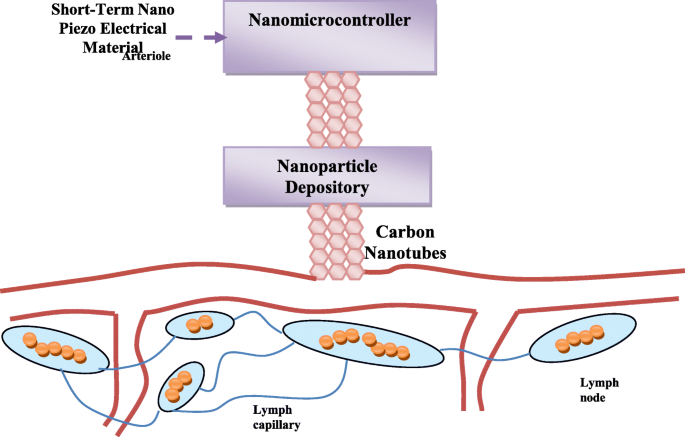
Allgemeine Struktur, die die Assoziation des vorgeschlagenen Wirkstoffsystems mit den infizierten Zellen zeigt
Dies ist kein zufälliger Prozess, sondern wird vom Nanocontroller unter Berücksichtigung mehrerer Aspekte und Logarithmen gesteuert, was eine effiziente und schnelle Lieferung der Nanopartikel gewährleistet. Um die Nanopartikel genau und schnell an die Krebszellen zu liefern, wird der kompressive binäre Suchalgorithmus verwendet [21]. Darüber hinaus werden Nanopartikel in unterschiedlichen Dichten abgegeben, damit das Medikament wirksamer wird. Diese Methoden und ihr Modus Operandi unter Verwendung des Nanocontrollers sind in Abb. 3 dargestellt. Die physikalische Struktur des Nanocontrollers ähnelt der der Nanopartikel, liegt jedoch in Form von Metall vor, sodass er elektrische Energie gewinnen kann für kurze Zeit während der Arbeit. Dieses Metall enthält eine drahtlose Antenne zusammen mit einem kleinen Speicher, der die Betriebscodes mit einer Nanopartikelverbindung zwischen dem Nanocontroller und dem Nanopartikelspeicher enthält. Das Nanopartikel-Repository enthält mehrere verschiedene Arten von Nanopartikeln. Das Öffnen und Schließen sowie die Öffnungsdauer des Nano-Gates werden gesteuert, um die Anzahl der abzugebenden Partikel anzupassen.
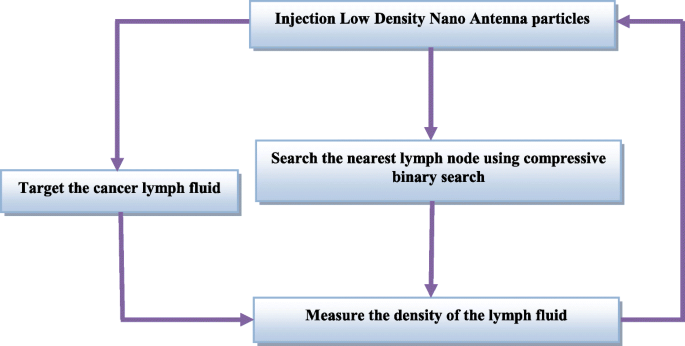
Sendeprozess der bekämpfenden Nanopartikel an Krebszellen
Eine Beschreibung der Natur der im vorgeschlagenen Arzneimittelsystem verwendeten Nanopartikel
Im nächsten Abschnitt wird die Natur der Nanopartikel diskutiert, die in dem vorgeschlagenen Wirkstoffabgabesystem verwendet werden. In dieser Arbeit wurden anaerobe Nanopartikel geringer Dichte verwendet, wie in einem früheren Bericht beschrieben [22].
Nanopartikel geringer Dichte
Betrachten Sie den Wirkstoffabgabeprozess von zusammengesetzten Nanopartikeln für Krebs als einen eindringenden Prozess in die Lymphflüssigkeit, bei dem ein Tumor von der Lymphflüssigkeit umgeben ist. Die Zusammensetzung des Melanoms ähnelt der Lymphflüssigkeit. Das vorgeschlagene analytische Modell basiert auf einem Nanoröhrensystem, das aus drei verschiedenen Arten von Nanopartikeln besteht. Die Nanopartikel werden in eine hochdichte Lymphflüssigkeit eingebracht. Wir können spezifische Nanopartikel des Festkörpers A in den sphärischen Polarkoordinaten als A . definieren = (ra, ϑa , φa), wobei ra die radiale Koordinate des Nanopartikels des Festkörpers A ist, ϑa ist die Zenithalkoordinate für das Nanopartikel von Festkörper A und φa ist die azimutale Koordinate für das Nanopartikel von Festkörper A. Die entsprechenden Koordinaten von Festkörper B sind B = (rb, ϑb, φb) und die entsprechenden Koordinaten des Festkörpers N sind N = (rn, ϑn, φn). Bedenken Sie, dass es zwei Eigenschaften des Lymphknotens gibt, nämlich zart und geschwollen, die von den Krebszellen des Hodgkin-Lymphoms beeinflusst werden. Der Lymphknoten mit empfindlicher Eigenschaft Tp kann als Tp (N ,t ); Dies bedeutet, dass der Wert von Tp in Verbindung mit der Flüssigkeit von Nanopartikeln von festem N mit der Zeit variiert. Betrachten wir nun, dass die Gesamtwirkung der zusammengesetzten Nanopartikel in der zarten Eigenschaft definiert ist als:
$$ \mathrm{Tpt}=\mathrm{Tp}\ \left(A,t\right)+\mathrm{Tp}\ \left(B,t\right)+.\dots\dots\dots\dots + \mathrm{Tp}\ \left(N,t\right) $$ (1)Betrachten Sie den gleichen Fall für die gequollene Eigenschaft, die wie folgt definiert werden könnte:
$$ \mathrm{Tst}=\mathrm{Ts}\ \left(A,t\right)+\mathrm{Ts}\ \left(B,t\right)+.\dots\dots\dots\dots + \mathrm{Ts}\ \left(N,t\right) $$ (2)Aus Gl. 1 und 2 kann die Änderungsrate beider Eigenschaften mit der Zeit bestimmt werden als:
$$ \frac{\partial\left(\mathrm{Tp}\left(A,t\right)\right)}{\partial t}+\frac{\partial\left(\mathrm{Tp}\left( B,t\right)\right)}{\partial t}+\dots\frac{\partial\left(\mathrm{Tp}\left(N,t\right)\right)}{\partial t}=\frac{\textrm{\partial Tp}(t)}{\textrm{\partial t}} $$ (3) $$ \frac{\partial\left(\textrm{Ts}\left(A,t\ rechts)\rechts)}{\partial t}+\frac{\partial\left(\textrm{Ts}\left(B,t\right)\right)}{\partial t}+\dots\frac{\ partielle \left(\textrm{Ts}\left(N,t\right)\right)}{\partial t}=\frac{\textrm{\partial Ts}(t)}{\partial t} $$ ( 4)Der Punkt in der Lymphflüssigkeit, der von einem Nanopartikel festem N besetzt werden kann, ist definiert als:
$$ {\textrm{Po}}_n=\textrm{Po}{\left(\textrm{po},t\right)}_n $$ (5)Nehmen wir an, ein Nanopartikel des festen N-Derivats der zarten Lymphflüssigkeit sei definiert als \( \frac{\partial {\left(\mathrm{Tp}\left(N,t\right)\right)}_{\ mathrm{po}}}{\partial t}\), dann wäre das zusammengesetzte Materialderivat der zarten Lymphflüssigkeit gleich:
$$ \frac{\partial {\left(\textrm{Tp}\left(A,t\right)\right)}_{\textrm{po}}}{\partial t}+\frac{\partial { \left(\textrm{Tp}\left(B,t\right)\right)}_{\textrm{po}}}{\partial t}+\dots \frac{\partial {\left(\textrm{ Tp}\left(N,t\right)\right)}_{\textrm{po}}}{\partial t}=\frac{\textrm{\partial Tp}{(t)}_{\textrm{ po}}}{\partial t} $$ (6) $$ \frac{\partial {\left(\textrm{Ts}\left(A,t\right)\right)}_{\textrm{po} }}{\partial t}+\frac{\partial {\left(\textrm{Ts}\left(B,t\right)\right)}_{\textrm{po}}}{\partial t}+ \dots \frac{\partial {\left(\textrm{Ts}\left(N,t\right)\right)}_{\textrm{po}}}{\partial t}=\frac{\textrm{ \partial Ts}{(t)}_{\textrm{po}}}{\partial t} $$ (7)Die entsprechenden Geschwindigkeitskomponenten des Festkörpers N werden als (v rn , v ϑn , v φn ). Dann wird die Fließgeschwindigkeit der Feststoffpartikel N unter Verwendung der Navier-Stokes-Gleichungen bei dynamischer Viskosität dν der Lymphflüssigkeit und p . dargestellt ist der Druck und ρ ist die Dichte der Lymphflüssigkeit wie folgt:
$$ \frac{\partial{v}_{\mathrm{rn}}}{\partial t}+{v}_{\mathrm{rn}}\frac{\partial{v}_{\mathrm{rn }}}{\mathrm{\partial rn}}+\frac{v_{\upvartheta\mathrm{n}}}{\mathrm{rn}}\frac{\partial{v}_{\mathrm{rn}} }{\mathrm{\partial\upvartheta n}}+\frac{v_{\upvarphi\mathrm{n}}}{\mathrm{rn}\\mathrm{sin}\upvartheta\mathrm{n}}\frac{ \partial{v}_{\mathrm{rn}}}{\mathrm{\partial\upvarphi n}}-\frac{v_{\upvartheta\mathrm{n}}^2}{\mathrm{rn}}- \frac{v_{\upvarphi\mathrm{n}}^2}{\mathrm{rn}}+\frac{1}{\rho}\frac{\partial p}{\mathrm{\partial rn}}- \mathrm{d}\upnu \left[\frac{1}{{\mathrm{rn}}^2}\frac{\partial }{\mathrm{\partial rn}}\left({\mathrm{rn} }^2\frac{\partial{v}_{\textrm{rn}}}{\textrm{\partial rn}}\right)+\frac{1}{{\textrm{rn}}^2\textrm {sin}\upvartheta\mathrm{n}}\frac{\partial }{\mathrm{\partial\upvartheta n}}\left(\mathrm{sin}\upvartheta\mathrm{n}\frac{\partial{v }_{\mathrm{rn}}}{\mathrm{\partial\upvartheta n}}\right)+\frac{1}{{\mathrm{rn}}^2{\sin}^2\upvartheta\mathrm {n}}\frac{\partial^2{v}_{\ma thrm{rn}}}{\partial{\upvarphi\mathrm{n}}^2}+-\frac{2{v}_{\mathrm{rn}}}{{\mathrm{rn}}^2} -\frac{2}{{\mathrm{rn}}^2\sin\upvartheta\mathrm{n}}\frac{\partial\left({v}_{\upvartheta\mathrm{n}}\mathrm{ sin}\upvartheta\mathrm{n}\right)}{\mathrm{\partial\upvartheta n}}-\frac{2}{{\mathrm{rn}}^2\mathrm{sin}\upvartheta\mathrm{ n}}\frac{\partial{v}_{\upvarphi\mathrm{n}}}{\partial_{\upvarphi\mathrm{n}}}\right]=0 $$ (8)Die dynamische Viskosität dν der Lymphflüssigkeit berechnet sich wie folgt:
$$ \mathrm{d}\upnu =\frac{\left[\frac{\partial {v}_{\mathrm{rn}}}{\partial t}+{v}_{\mathrm{rn}} \frac{\partial{v}_{\mathrm{rn}}}{\mathrm{\partial rn}}+\frac{v_{\upvartheta\mathrm{n}}\partial{v}_{\mathrm{ rn}}}{\mathrm{rn}\\mathrm{\partial\upvartheta n}}+\frac{v_{\upvarphi\mathrm{n}}}{\mathrm{rn}\\mathrm{sin}\upvartheta \mathrm{n}}\frac{\partial{v}_{\mathrm{rn}}}{\mathrm{\partial\upvarphi n}}-\frac{v_{\upvartheta\mathrm{n}}^2 }{\mathrm{rn}}-\frac{v_{\upvarphi\mathrm{n}}^2}{\mathrm{rn}}+\frac{1\\partial p}{\uprho\partial\mathrm{ rn}}\right]}{\left[\frac{1}{{\textrm{rn}}^2}\frac{\partial }{\textrm{\partial rn}}\left({\textrm{rn }}^2\frac{\partial{v}_{\textrm{rn}}}{\textrm{\partial rn}}\right)+\frac{1}{{\textrm{rn}}^2\ mathrm{sin}\upvartheta\mathrm{n}}\frac{\partial }{\mathrm{\partial\upvartheta n}}\left(\mathrm{sin}\upvartheta\mathrm{n}\frac{\partial{ v}_{\mathrm{rn}}}{\mathrm{\partial\upvartheta n}}\right)+\frac{1}{{\mathrm{rn}}^2{\sin}^2\upvartheta\ mathrm{n}}\frac{\partial ^2{v}_{\mathrm{rn}}}{\partial {\upvarphi\mathrm{n}}^2}+-\frac{2{v}_{\mathrm{rn}}}{{\ mathrm{rn}}^2}-\frac{2}{{\mathrm{rn}}^2\sin\upvartheta\mathrm{n}}\frac{\partial\left({v}_{\upvartheta\ mathrm{n}}\mathrm{sin}\upvartheta\mathrm{n}\right)}{\mathrm{\partial\upvartheta n}}-\frac{2}{{\mathrm{rn}}^2\mathrm {sin}\upvartheta\mathrm{n}}\frac{\partial{v}_{\upvarphi\mathrm{n}}}{\partial_{\upvarphi\mathrm{n}}}\right]} $$ ( 9)Die Navier-Stokes-Gleichungen der Festkörper A und B könnten als Gl. 8 und 9. Somit ist Gl. 9 könnte wie folgt dargestellt werden:
$$ \mathrm{d}\upnu =\frac{\left[\frac{\partial {v}_{\mathrm{rn}}}{\partial t}+{v}_{\mathrm{rn}} \frac{\partial{v}_{\mathrm{rn}}}{\mathrm{\partial rn}}+\frac{v_{\upvartheta\mathrm{n}}}{\mathrm{rn}}\frac {\partial{v}_{\mathrm{rn}}}{\mathrm{\partial\upvartheta n}}+\frac{v_{\upvarphi\mathrm{n}}}{\mathrm{rn}\ \mathrm {sin}\upvartheta\mathrm{n}}\frac{\partial{v}_{\mathrm{rn}}}{\mathrm{\partial\upvarphi n}}-\frac{v_{\upvartheta\mathrm{ n}}^2}{\mathrm{rn}}-\frac{v_{\upvarphi\mathrm{n}}^2}{\mathrm{rn}}+\frac{1}{\rho}\frac{ \partial p}{\mathrm{\partial rn}}\right]}{\left[\frac{1}{{\mathrm{rn}}^2}\frac{\partial }{\mathrm{\partial rn }}\left({\textrm{rn}}^2\frac{\partial{v}_{\textrm{rn}}}{\textrm{\partial rn}}\right)+\frac{1}{ {\mathrm{rn}}^2\mathrm{sin}\upvartheta \mathrm{n}}\frac{\partial }{\mathrm{\partial\upvartheta n}}\left(\mathrm{sin}\upvartheta\ mathrm{n}\frac{\partial{\mathrm{v}}_{\mathrm{rn}}}{\mathrm{\partial\upvartheta n}}\right)+\frac{1}{{\mathrm{ rn}}^2{\sin}^2\upvartheta\math rm{n}}\frac{\partial^2{\mathrm{v}}_{\mathrm{rn}}}{\partial {\upvarphi\mathrm{n}}^2}-\frac{2{\ mathrm{v}}_{\mathrm{rn}}}{{\mathrm{rn}}^2}-\frac{2}{{\mathrm{rn}}^2\sin\upvartheta\mathrm{n} }\frac{\partial\left({\mathrm{v}}_{\upvartheta\mathrm{n}}\mathrm{sin}\upvartheta\mathrm{n}\right)}{\mathrm{\partial\upvartheta n}}-\frac{2}{{\mathrm{rn}}^2\mathrm{sin}\upvartheta\mathrm{n}}\frac{\partial{\mathrm{v}}_{\upvarphi\mathrm {n}}}{\partial_{\upvarphi\mathrm{n}}}\right]}=\frac{\left[\frac{\partial {\mathrm{v}}_{\mathrm{ra}}} {\mathrm{\mathrm{v}}+{\mathrm{v}}_{\mathrm{ra}}\frac{\partial{\mathrm{v}}_{\mathrm{ra}}}{\mathrm{ \partial ra}}+\frac{{\mathrm{v}}_{\upvartheta \mathrm{a}}}{\mathrm{ra}}\frac{\partial {\mathrm{v}}_{\mathrm {ra}}}{\mathrm{\partial\upvartheta a}}+\frac{{\mathrm{v}}_{\upvarphi\mathrm{a}}}{\mathrm{ra}\ \mathrm{sin} \upvartheta \mathrm{a}}\frac{\partial {\mathrm{v}}_{\mathrm{ra}}}{\mathrm{\partial \upvarphi a}}-\frac{{\mathrm{v} }_{\upvartheta\mathrm{a}}^2}{\mathrm{ra}}- \frac{{\mathrm{v}}_{\upvarphi\mathrm{a}}^2}{\mathrm{ra}}+\frac{1}{\uprho}\frac{\mathrm{\partial p} }{\mathrm{\partial ra}}\right]}{\left[\frac{1}{{\mathrm{ra}}^2}\frac{\partial }{\mathrm{\partial ra}}\ links({\mathrm{ra}}^2\frac{\partial{v}_{\mathrm{ra}}}{\mathrm{\partial ra}}\right)+\frac{1}{{\mathrm {ra}}^2\mathrm{sin}\upvartheta \mathrm{a}}\frac{\partial }{\mathrm{\partial \upvartheta a}}\left(\mathrm{sin}\upvartheta \mathrm{a }\frac{\partial{v}_{\textrm{ra}}}{\textrm{\partial\upvartheta a}}\right)+\frac{1}{{\textrm{ra}}^2{\ sin}^2\upvartheta\mathrm{a}}\frac{\partial^2{v}_{\mathrm{ra}}}{\partial{\upvarphi\mathrm{a}}^2}-\frac{ 2{v}_{\mathrm{ra}}}{{\mathrm{ra}}^2}-\frac{2}{{\mathrm{ra}}^2\sin\upvartheta\mathrm{a}} \frac{\partial\left({v}_{\upvartheta\mathrm{a}}\mathrm{sin}\upvartheta\mathrm{a}\right)}{\mathrm{\partial\upvartheta a}}-\ frac{2}{{\mathrm{ra}}^2\mathrm{sin}\upvartheta\mathrm{a}}\frac{\partial{v}_{\upvarphi\mathrm{a}}}{\partial_{ \upvarphi\mathrm{a}}}\right]}=\frac{ \left[\frac{\partial{v}_{\textrm{rb}}}{\partial t}+{v}_{\textrm{rb}}\frac{\partial{v}_{\textrm{ rb}}}{\mathrm{\partialrb}}+\frac{v_{\upvartheta\mathrm{b}}}{\mathrm{rb}}\frac{\partial{v}_{\mathrm{rb} }}{\mathrm{\partial\upvartheta b}}+\frac{v_{\upvarphi\mathrm{b}}}{\mathrm{rb}\\mathrm{sin}\upvartheta\mathrm{b}}\frac {\partial{v}_{\mathrm{rb}}}{\mathrm{\partial\upvarphi b}}-\frac{v_{\upvartheta\mathrm{b}}^2}{\mathrm{rb}} -\frac{v_{\upvarphi\mathrm{b}}^2}{\mathrm{rb}}+\frac{1}{\rho}\frac{\partial p}{\mathrm{\partial rb}} \right]}{\left[\frac{1}{{\textrm{rb}}^2}\frac{\partial }{\textrm{\partialrb}}\left({\textrm{rb}}^ 2\frac{\partial{v}_{\textrm{rb}}}{\textrm{\partialrb}}\right)+\frac{1}{{\textrm{rb}}^2\textrm{sin }\upvartheta\mathrm{b}}\frac{\partial }{\mathrm{\partial\upvartheta b}}\left(\mathrm{sin}\upvartheta\mathrm{b}\frac{\partial{v}_ {\mathrm{rb}}}{\mathrm{\partial\upvartheta b}}\right)+\frac{1}{{\mathrm{rb}}^2{\sin}^2\upvartheta \mathrm{b }}\frac{\partial^2{v}_{\mathrm{ rb}}}{\partial {\upvarphi\mathrm{b}}^2}-\frac{2{v}_{\mathrm{rb}}}{{\mathrm{rb}}^2}-\frac {2}{{\mathrm{rb}}^2\sin\upvartheta\mathrm{b}}\frac{\partial\left({v}_{\upvartheta\mathrm{b}}\mathrm{sin}\ upvartheta \mathrm{b}\right)}{\mathrm{\partial \upvartheta b}}-\frac{2}{{\mathrm{rb}}^2\mathrm{sin}\upvartheta \mathrm{b}} \frac{\partial{v}_{\upvarphi\mathrm{b}}}{\partial_{\upvarphi\mathrm{b}}}\right]} $$ (10)Die Partikel haben Nano-Dimensionen; daher wären ihre Radien sehr klein, und der Einfachheit halber gilt Gl. 10 wird wie folgt dargestellt:
$$ \mathrm{d}\upnu =\left[\frac{v_{\upvartheta\mathrm{n}}}{\mathrm{rn}}\frac{\partial {v}_{\mathrm{rn}} }{\mathrm{\partial\upvartheta n}}+\frac{v_{\upvarphi\mathrm{n}}}{\mathrm{rn}\\mathrm{sin}\upvartheta\mathrm{n}}\frac{ \partial{v}_{\mathrm{rn}}}{\mathrm{\partial\upvarphi n}}-\frac{v_{\upvartheta\mathrm{n}}^2}{\mathrm{rn}}- \frac{v_{\upvarphi\mathrm{n}}^2}{\mathrm{rn}}+\frac{1}{\rho}\frac{\partial p}{\mathrm{\partial rn}}\ rechts]/\links[\frac{1}{{\textrm{rn}}^2}\frac{\partial }{\textrm{\partial rn}}\left({\textrm{rn}}^2\ frac{\partial{v}_{\textrm{rn}}}{\textrm{\partial rn}}\right)+\frac{1}{{\textrm{rn}}^2\textrm{sin}\ upvartheta \mathrm{n}}\frac{\partial }{\mathrm{\partial \upvartheta n}}\left(\mathrm{sin}\upvartheta \mathrm{n}\frac{\partial {\mathrm{v} }_{\mathrm{rn}}}{\mathrm{\partial\upvartheta n}}\right)+\frac{1}{{\mathrm{rn}}^2{\sin}^2\upvartheta\mathrm {n}}\frac{\partial^2{\mathrm{v}}_{\mathrm{rn}}}{\partial {\upvarphi\mathrm{n}}^2}-\frac{2{\mathrm {v}}_{\mathrm{rn}}}{{\math rm{rn}}^2}-\frac{2}{{\mathrm{rn}}^2\sin\upvartheta\mathrm{n}}\frac{\partial\left({\mathrm{v}}_ {\upvartheta\mathrm{n}}\mathrm{sin}\upvartheta\mathrm{n}\right)}{\mathrm{\partial\upvartheta n}}-\frac{2}{{\mathrm{rn}} ^2\mathrm{sin}\upvartheta\mathrm{n}}\frac{\partial {\mathrm{v}}_{\upvarphi\mathrm{n}}}{\partial_{\upvarphi\mathrm{n}} }\right]=\left[\frac{{\mathrm{v}}_{\upvartheta\mathrm{a}}}{\mathrm{ra}}\frac{\partial {\mathrm{v}}_{ \mathrm{ra}}}{\mathrm{\partial \upvartheta a}}}+\frac{{\mathrm{v}}_{\upvarphi\mathrm{a}}}{\mathrm{ra}\ \mathrm{ sin}\upvartheta\mathrm{a}}\frac{\partial{\mathrm{v}}_{\mathrm{ra}}}{\mathrm{\partial\upvarphi a}}-\frac{{\mathrm{ v}}_{\upvartheta\mathrm{a}}^2}{\mathrm{ra}}-\frac{{\mathrm{v}}_{\upvarphi\mathrm{a}}^2}{\mathrm {ra}}+\frac{1}{\uprho}\frac{\mathrm{\partial p}}{\mathrm{\partial ra}}\right]/\left[\frac{1}{{\mathrm {ra}}^2}\frac{\partial }{\mathrm{\partial ra}}\left({\mathrm{ra}}^2\frac{\partial {\mathrm{v}}_{\mathrm {ra}}}{\mathrm{\partialra}}\rig ht)+\frac{1}{{\mathrm{ra}}^2\mathrm{sin}\upvartheta\mathrm{a}}\frac{\partial}{\mathrm{\partial\upvartheta a}}\left (\mathrm{sin}\upvartheta\mathrm{a}\frac{\partial{\mathrm{v}}_{\mathrm{ra}}}{\mathrm{\partial\upvartheta a}}\right)+\ frac{1}{{\mathrm{ra}}^2{\sin}^2\upvartheta\mathrm{a}}\frac{\partial^2{\mathrm{v}}_{\mathrm{ra}} }{\partial{\upvarphi\mathrm{a}}^2}-\frac{2{\mathrm{v}}_{\mathrm{ra}}}{{\mathrm{ra}}^2}-\ frac{2}{{\mathrm{ra}}^2\sin\upvartheta\mathrm{a}}\frac{\partial\left({\mathrm{v}}_{\upvartheta\mathrm{a}}\ mathrm{sin}\upvartheta\mathrm{a}\right)}{\mathrm{\partial\upvartheta a}}-\frac{2}{{\mathrm{ra}}^2\mathrm{sin}\upvartheta\ mathrm{a}}\frac{\partial{v}_{\upvarphi\mathrm{a}}}{\partial_{\upvarphi\mathrm{a}}}\right]=\left[\frac{v_{\ upvartheta \mathrm{b}}}{\mathrm{rb}}\frac{\partial {v}_{\mathrm{rb}}}{\mathrm{\partial \upvartheta b}}+\frac{v_{\ upvarphi \mathrm{b}}}{\mathrm{rb}\ \mathrm{sin}\upvartheta \mathrm{b}}\frac{\partial{v}_{\mathrm{rb}}}{\mathrm{\ teilweise \ upvarphi b}}-\frac{v_{\upvartheta\mathrm{b}}^2}{\mathrm{rb}}-\frac{v_{\upvarphi\mathrm{b}}^2}{\mathrm{rb }}+\frac{1}{\rho}\frac{\partial p}{\textrm{\partial rb}}\right]/\left[\frac{1}{{\textrm{rb}}^2 }\frac{\partial }{\textrm{\partialrb}}\left({\textrm{rb}}^2\frac{\partial{v}_{\textrm{rb}}}{\textrm{\ partielles rb}}\right)+\frac{1}{{\mathrm{rb}}^2\mathrm{sin}\upvartheta \mathrm{b}}\frac{\partial }{\mathrm{\partial\upvartheta b}}\left(\mathrm{sin}\upvartheta\mathrm{b}\frac{\partial{v}_{\mathrm{rb}}}{\mathrm{\partial\upvartheta b}}\right)+ \frac{1}{{\mathrm{rb}}^2{\sin}^2\upvartheta \mathrm{b}}\frac{\partial^2{v}_{\mathrm{rb}}}{\ partielle {\upvarphi\mathrm{b}}^2}-\frac{2{v}_{\mathrm{rb}}}{{\mathrm{rb}}^2}-\frac{2}{{\ mathrm{rb}}^2\sin\upvartheta\mathrm{b}}\frac{\partial\left({v}_{\upvartheta\mathrm{b}}\mathrm{sin}\upvartheta\mathrm{b} \right)}{\mathrm{\partial\upvartheta b}}-\frac{2}{{\mathrm{rb}}^2\mathrm{sin}\upvartheta\mathrm{b}}\frac{\partial { v}_{\upvarphi\mathrm{b}}}{\partial _{\upvarphi\mathrm{b}}}\right] $$ (11)Gleichung 11 könnte wie folgt dargestellt werden:
$$ \mathrm{d}\upnu =\mathrm{rn}\left[{v}_{\upvartheta \mathrm{n}}\frac{\partial{v}_{\mathrm{rn}}}{\ mathrm{\partial\upvartheta n}}+\frac{v_{\upvarphi\mathrm{n}}}{\\mathrm{sin}\upvartheta\mathrm{n}}\frac{\partial{v}_{\ mathrm{rn}}}{\mathrm{\partial\upvarphi n}}-{v}_{\upvartheta\mathrm{n}}^2-{v}_{\upvarphi\mathrm{n}}^2+ \frac{\mathrm{rn}}{\rho}\frac{\partial p}{\mathrm{\partial rn}}\right]/\left[\frac{\partial }{\mathrm{\partial rn} }\left({\mathrm{rn}}^2\frac{\partial {v}_{\mathrm{rn}}}{\mathrm{\partial rn}}\right)+\frac{1}{\ mathrm{sin}\upvartheta\mathrm{n}}\frac{\partial }{\mathrm{\partial\upvartheta n}}\left(\mathrm{sin}\upvartheta\mathrm{n}\frac{\partial{ v}_{\mathrm{rn}}}{\mathrm{\partial\upvartheta n}}\right)+\frac{1}{\sin^2\upvartheta\mathrm{n}}\frac{\partial^ 2{v}_{\mathrm{rn}}}{\partial{\upvarphi\mathrm{n}}^2}-2{v}_{\mathrm{rn}}-\frac{2}{\sin \upvartheta \mathrm{n}}\frac{\partial \left({v}_{\upvartheta \mathrm{n}}\mathrm{sin}\upvartheta \mathrm{n}\right)}{\mathrm{\ Par tial \upvartheta n}}-\frac{2}{\mathrm{sin}\upvartheta \mathrm{n}}\frac{\partial{v}_{\upvarphi\mathrm{n}}}{\partial_{\ upvarphi \mathrm{n}}}\right]=\mathrm{ra}\left[{v}_{\upvartheta\mathrm{a}}\frac{\partial {v}_{\mathrm{ra}}} {\mathrm{\partial\upvartheta a}}+\frac{v_{\upvarphi\mathrm{a}}}{\\mathrm{sin}\upvartheta\mathrm{a}}\frac{\partial{v}_ {\mathrm{ra}}}{\mathrm{\partial\upvarphi a}}-{v}_{\upvartheta\mathrm{a}}^2-{v}_{\upvarphi\mathrm{a}}^ 2+\frac{\mathrm{ra}}{\rho}\frac{\partial p}{\mathrm{\partial ra}}\right]/\left[\frac{\partial }{\mathrm{\partial ra}}\left({\mathrm{ra}}^2\frac{\partial{v}_{\mathrm{ra}}}{\mathrm{\partial ra}}\right)+\frac{1} {\mathrm{sin}\upvartheta\mathrm{a}}\frac{\partial }{\mathrm{\partial\upvartheta a}}\left(\mathrm{sin}\upvartheta\mathrm{a}\frac{\ partielle {v}_{\mathrm{ra}}}{\mathrm{\partial\upvartheta a}}\right)+\frac{1}{\sin^2\upvartheta\mathrm{a}}\frac{\ partiell^2{v}_{\mathrm{ra}}}{\partial{\upvarphi\mathrm{a}}^2}-2{v}_{\mathrm{ra}}-\frac{2}{ \Sünde \upvartheta \mathrm{a}}\frac{\partial \left({v}_{\upvartheta \mathrm{a}}\mathrm{sin}\upvartheta \mathrm{a}\right)}{\mathrm{\ partieller \upvartheta a}}-\frac{2}{\mathrm{sin}\upvartheta \mathrm{a}}\frac{\partial{v}_{\upvarphi\mathrm{a}}}{\partial_{\ upvarphi \mathrm{a}}}\right]=\mathrm{rb}\left[{v}_{\upvartheta\mathrm{b}}\frac{\partial {v}_{\mathrm{rb}}} {\mathrm{\partial\upvartheta b}}+\frac{v_{\upvarphi\mathrm{b}}}{\\mathrm{sin}\upvartheta\mathrm{b}}\frac{\partial{v}_ {\mathrm{rb}}}{\mathrm{\partial\upvarphi b}}-{v}_{\upvartheta\mathrm{b}}^2-{v}_{\upvarphi\mathrm{b}}^ 2+\frac{\mathrm{rb}}{\rho}\frac{\partial p}{\mathrm{\partial rb}}\right]/\left[\frac{\partial }{\mathrm{\partial rb}}\left({\textrm{rb}}^2\frac{\partial{v}_{\textrm{rb}}}{\textrm{\textrm{rb}}\right)+\frac{1} {\mathrm{sin}\upvartheta\mathrm{b}}\frac{\partial}{\mathrm{\partial\upvartheta b}}\left(\mathrm{sin}\upvartheta\mathrm{b}\frac{\ partielle {v}_{\textrm{rb}}}{\textrm{\partial\upvartheta b}}\right)+\frac{1}{\si n^2\upvartheta\mathrm{b}}\frac{\partial^2{v}_{\mathrm{rb}}}{\partial {\upvarphi\mathrm{b}}^2}-2{v} _{\mathrm{rb}}-\frac{2}{\sin\upvartheta\mathrm{b}}\frac{\partial\left({v}_{\upvartheta\mathrm{b}}\mathrm{sin }\upvartheta \mathrm{b}\right)}{\mathrm{\partial \upvartheta b}}-\frac{2}{\mathrm{sin}\upvartheta \mathrm{b}}\frac{\partial {v }_{\upvarphi\mathrm{b}}}{\partial_{\upvarphi\mathrm{b}}}\right] $$ (12)Zwischen den Radien der Nanopartikel und der krebsbedingten Lymphviskosität besteht ein direkter Zusammenhang. Wenn die Lymphe zu statisch und zähflüssig wird, kann sie ihre Funktion, die zirkulieren und Giftstoffe säubert und hilft, Krebs zu bekämpfen, nicht richtig erfüllen. Wenn die Nanopartikelgröße kleiner ist, können die lymphatischen Krebszellen leicht abgetötet werden. Um den Transport der Gesamtmenge der zusammengesetzten Nanopartikel zu beschreiben, verwenden wir die Kontinuitätsgleichung und nehmen die drei Nanopartikel der Festkörper A, B und N wie folgt an:
$$ \frac{1}{{\mathrm{ra}}^2}\frac{\partial }{\mathrm{\partial ra}}\left({\mathrm{ra}}^2{v}_{ \mathrm{ra}}\right)+\frac{1}{\mathrm{ra}\ \mathrm{sin}\upvartheta \mathrm{a}}\frac{\partial }{\mathrm{\partial \upvartheta a }}\left(\sin {\upvartheta\mathrm{v}}_{\upvartheta\mathrm{a}}\right)+\frac{1}{\mathrm{ra}\ \mathrm{sin}\upvartheta\ mathrm{a}}\frac{\partial{v}_{\upvarphi\mathrm{a}}}{\mathrm{\partial\upvarphi a}}}+\frac{1}{{\mathrm{rb}}^ 2}\frac{\partial }{\textrm{\partial rb}}\left({\textrm{rb}}^2{v}_{\textrm{rb}}\right)+\frac{1}{ \mathrm{rb}\ \mathrm{sin}\upvartheta \mathrm{b}}\frac{\partial }{\mathrm{\partial \upvartheta b}}\left(\sin {\upvartheta v}_{\upvartheta \mathrm{b}}\right)+\frac{1}{\mathrm{rb}\ \mathrm{sin}\upvartheta \mathrm{b}}\frac{\partial{v}_{\upvarphi \mathrm{ b}}}{\mathrm{\partial\upvarphi b}}+\frac{1}{{\mathrm{rn}}^2}\frac{\partial}{\mathrm{\partial rn}}\left( {\mathrm{rn}}^2{v}_{\mathrm{rn}}\right)+\frac{1}{\mathrm{rn}\ \mathrm{sin}\upvartheta \mathrm{n}}\ frac{\parti al }{\mathrm{\partial\upvartheta n}}\left(\sin {\upvartheta v}_{\upvartheta\mathrm{n}}\right)+\frac{1}{\mathrm{rn}\\ mathrm{sin}\upvartheta\mathrm{n}}\frac{\partial{v}_{\upvarphi\mathrm{n}}}{\mathrm{\partial\upvarphi n}}=0 $$ (13)Die dynamische Flüssigkeitsviskosität könnte aus der folgenden Gleichung [23] bestimmt werden:
$$ \mathrm{Vs}=\frac{2}{9}\frac{r^2g\ \left(\uprho\mathrm{p}-\uprho\mathrm{f}\right)}{\mathrm{dv }} $$ (14)where Vs is the particles’ settling velocity (m/s), r is the Stokes radius of the particle (m), g is the gravitational acceleration (m/s 2 ), ρp is the density of the particles (kg/m 3 ), ρf is the density of the fluid (kg/m 3 ), and dv is the (dynamic) fluid viscosity (Pa·s). The lymph fluid is slightly heavier than water (lymph density = 1019 kg/m 3 , water density = 998.28 kg/m 3 at 20 °C). As a reference value, we consider the dynamic viscosity of the water to be 1.002 × 10 –3 kg m –1 s –1 ).
Dynamic viscosity is the measurement of the fluid’s internal resistance to flow, while kinematic viscosity refers to the ratio of dynamic viscosity to density. The effect of all the nanoparticles on the fluid viscosity is represented as follows:
$$ \mathrm{dv}=\frac{2\mathrm{g}}{9}\left[\frac{{\mathrm{ra}}^2\left(\uprho \mathrm{a}-\uprho \mathrm{f}\right)}{\mathrm{vsa}}+\frac{{\mathrm{rb}}^2\left(\uprho \mathrm{b}-\uprho \mathrm{f}\right)}{\mathrm{vsb}}+\frac{{\mathrm{rn}}^2\left(\uprho \mathrm{n}-\uprho \mathrm{f}\right)}{\mathrm{vn}}\right] $$ (15)By comparing Eq. 12 and Eq. 15, the following equation could be emerged:
$$ \left|\frac{2\mathrm{g}}{9}\left[\frac{{\mathrm{ra}}^2\left(\uprho \mathrm{a}-\uprho \mathrm{f}\right)}{\mathrm{vsa}}+\frac{{\mathrm{rb}}^2\left(\uprho \mathrm{b}-\uprho \mathrm{f}\right)}{\mathrm{vsb}}+\frac{{\mathrm{rn}}^2\left(\uprho \mathrm{n}-\uprho \mathrm{f}\right)}{\mathrm{vn}}\right]\right|=\mathrm{rn}\left[{v}_{\upvartheta \mathrm{n}}\frac{\partial {v}_{\mathrm{rn}}}{\mathrm{\partial \upvartheta n}}+\frac{v_{\upvarphi \mathrm{n}}}{\ \mathrm{sin}\upvartheta \mathrm{n}}\frac{\partial {v}_{\mathrm{rn}}}{\mathrm{\partial \upvarphi n}}-{v}_{\upvartheta \mathrm{n}}^2-{v}_{\upvarphi \mathrm{n}}^2+\frac{\mathrm{rn}}{\uprho \mathrm{f}}\frac{\mathrm{\partial p}}{\mathrm{\partial rn}}\right]/\left[\frac{\partial }{\mathrm{\partial rn}}\left({\mathrm{rn}}^2\frac{\partial {v}_{\mathrm{rn}}}{\mathrm{\partial rn}}\right)+\frac{1}{\mathrm{sin}\upvartheta \mathrm{n}}\frac{\partial }{\mathrm{\partial \upvartheta n}}\left(\mathrm{sin}\upvartheta \mathrm{n}\frac{\partial {v}_{\mathrm{rn}}}{\mathrm{\partial \upvartheta n}}\right)+\frac{1}{\sin^2\upvartheta \mathrm{n}}\frac{\partial^2{v}_{\mathrm{rn}}}{\partial {\upvarphi \mathrm{n}}^2}-2{v}_{\mathrm{rn}}-\frac{2}{\sin \upvartheta \mathrm{n}}\frac{\partial \left({v}_{\upvartheta \mathrm{n}}\mathrm{sin}\upvartheta \mathrm{n}\right)}{\mathrm{\partial \upvartheta n}}-\frac{2}{\mathrm{sin}\upvartheta \mathrm{n}}\frac{\partial {v}_{\upvarphi \mathrm{n}}}{\partial_{\upvarphi \mathrm{n}}}\right]=\mathrm{ra}\left[{v}_{\upvartheta \mathrm{a}}\frac{\partial {v}_{\mathrm{ra}}}{\mathrm{\partial \upvartheta a}}+\frac{v_{\upvarphi \mathrm{a}}}{\ \mathrm{sin}\upvartheta \mathrm{a}}\frac{\partial {v}_{\mathrm{ra}}}{\mathrm{\partial \upvarphi a}}-{v}_{\upvartheta \mathrm{a}}^2-{v}_{\upvarphi \mathrm{a}}^2+\frac{\mathrm{ra}}{\uprho \mathrm{f}}\frac{\partial p}{\mathrm{\partial ra}}\right]/\left[\frac{\partial }{\mathrm{\partial ra}}\left({\mathrm{ra}}^2\frac{\partial {v}_{\mathrm{ra}}}{\mathrm{\partial ra}}\right)+\frac{1}{\mathrm{sin}\upvartheta \mathrm{a}}\frac{\partial }{\mathrm{\partial \upvartheta a}}\left(\mathrm{sin}\upvartheta \mathrm{a}\frac{\partial {v}_{\mathrm{ra}}}{\mathrm{\partial \upvartheta a}}\right)+\frac{1}{\sin^2\upvartheta \mathrm{a}}\frac{\partial^2{v}_{\mathrm{ra}}}{\partial {\upvarphi \mathrm{a}}^2}-2{v}_{\mathrm{ra}}-\frac{2}{\sin \upvartheta \mathrm{a}}\frac{\partial \left({v}_{\upvartheta \mathrm{a}}\mathrm{sin}\upvartheta \mathrm{a}\right)}{\mathrm{\partial \upvartheta a}}-\frac{2}{\mathrm{sin}\upvartheta \mathrm{a}}\frac{\partial {v}_{\upvarphi \mathrm{a}}}{\partial_{\upvarphi \mathrm{a}}}\right]=\mathrm{rb}\left[{v}_{\upvartheta \mathrm{b}}\frac{\partial {v}_{\mathrm{rb}}}{\mathrm{\partial \upvartheta b}}+\frac{v_{\upvarphi \mathrm{b}}}{\ \mathrm{sin}\upvartheta \mathrm{b}}\frac{\partial {v}_{\mathrm{rb}}}{\mathrm{\partial \upvarphi b}}-{v}_{\upvartheta \mathrm{b}}^2-{v}_{\upvarphi \mathrm{b}}^2+\frac{\mathrm{rb}}{\uprho \mathrm{f}}\frac{\partial p}{\mathrm{\partial rb}}\right]/\left[\frac{\partial }{\mathrm{\partial rb}}\left({\mathrm{rb}}^2\frac{\partial {v}_{\mathrm{rb}}}{\mathrm{\partial rb}}\right)+\frac{1}{\mathrm{sin}\upvartheta \mathrm{b}}\frac{\partial }{\mathrm{\partial \upvartheta b}}\left(\mathrm{sin}\upvartheta \mathrm{b}\frac{\partial {v}_{\mathrm{rb}}}{\mathrm{\partial \upvartheta b}}\right)+\frac{1}{\sin^2\upvartheta \mathrm{b}}\frac{\partial^2{v}_{\mathrm{rb}}}{\partial {\upvarphi \mathrm{b}}^2}-2{v}_{\mathrm{rb}}-\frac{2}{\sin \upvartheta \mathrm{b}}\frac{\partial \left({v}_{\upvartheta \mathrm{b}}\mathrm{sin}\upvartheta \mathrm{b}\right)}{\mathrm{\partial \upvartheta b}}-\frac{2}{\mathrm{sin}\upvartheta \mathrm{b}}\frac{\partial {v}_{\upvarphi \mathrm{b}}}{\partial_{\upvarphi \mathrm{b}}}\right] $$ (16)Equation 16 depicts the relationship between the density of the lymph fluid, the density of the nanoparticles of the compound drug system, and the radius of the nanoparticles. There is a positive correlation between the density of the lymph fluid and the density of the nanoparticles. The smaller the density and radius of nanoparticles, the lesser the density of the fluid will be. As established earlier, the decrease in the density of the lymph fluid leads to its inability to reproduce and reduce the ferocity of the disease. It can, therefore, be concluded that the tumor can be cured by minimizing the size of nanoparticles. These particles can reach in the range of up to 0.1 nm (i.e., Angstrom or picometer range). The particles of this size can act as the nucleus of drug delivery in this drug system. Equation 16 shows that radii of the nanoparticles in the proposed drug system are related to the effectiveness of the delivery system. Much lower sized nanoparticles can reduce the density of lymph fluid and the spread of the disease.
Nanoparticles with Nanoantennas
This study used the nanoparticle described in an earlier report [22] as an emissary with a nano-microcontroller. In the system, the proposed transmission distance is very small and compatible with the composition of nanoparticles. Thus, the middle gap can be neglected in mid-distance and is symbolized by C d . Further, R a und X a are the real part and the imaginary part of the anaerobic impedance. After neglecting the load of the intercellular space between the nanoparticles and the nano-microcontroller, R a und X a can be calculated as follows [22]:
$$ {R}_a=\frac{r_{a0}}{1+{C}_d{w}_a\left(2{x}_{a0}+{C}_d\left({r_{a0}}^2+{x_{a0}}^2\right){w}_a\right)} $$ (17) $$ {X}_a=\frac{x_0-{C}_d\left({r_{a0}}^2+{x_{a0}}^2\right){w}_a}{1+{C}_d{w}_a\left(2{x}_{a0}+{C}_d\left({r_{a0}}^2+{x_{a0}}^2\right){w}_a\right)} $$ (18)Thus, the load resistance value of nanotubes can be predicted as in the following equation:
$$ {r}_l=\frac{g^2R}{g^2-2 gSX{\varepsilon}_L\omega +{S}^2\left({R}^2+{X}^2\right){\varepsilon_L}^2{\omega}^2} $$ (19)wo ε L is the permittivity of the loading material, g is the size of the gap, and S is the effective cross-section area of the gap. In order to simplify the equation, the value of g 2 can be neglected as it is too low and the final equation can be rewritten as follows:
$$ {r}_l=\frac{g^2R}{-2 gSX{\varepsilon}_L\omega +{S}^2\left({R}^2+{X}^2\right){\varepsilon_L}^2{\omega}^2} $$ (20)Then,
$$ {r}_l=\frac{g^2R}{S{\varepsilon}_L\omega \left(-2 gX+S\left({R}^2+{X}^2\right){\varepsilon}_L\omega \right)} $$ (21)The optical nano-photo concept can be used as an effective tool for interpreting and predicting these effects to design and improve nanoscale parameters and increase the nano-sensitivity to serve better as a single molecular sensor. Nanoantenna may provide optimal performance in terms of sensitivity, efficiency, and bandwidth in the process. The next section presents the concept of searching the cancerous lymph nodes using compressive binary search algorithm.
Searching for the Target Lymphatic Nodes Using Compressive Binary Search
In order for nanoparticles to reach the cancerous cells in a fast and efficient manner, we applied compressive binary search by the nano-microcontroller. The guided nanoparticles follow a specific path to quickly reach the target. This movement is based on the information obtained from the “exploratory nanoparticles.” Assume that the target lymph node, Tf, has exactly one nonzero entry, where the location of the lymph node is unknown. The algorithm divides mt measurements into a total of St stages, where St refers to the stages of the lymph nodes. The measurements are more than one for all the stages of the lymph nodes, which is necessary for the algorithm to be executed until completion. Based on this measurement, the algorithm decides between going left or right, until the nanoparticles reach the target, the cancerous lymph node.
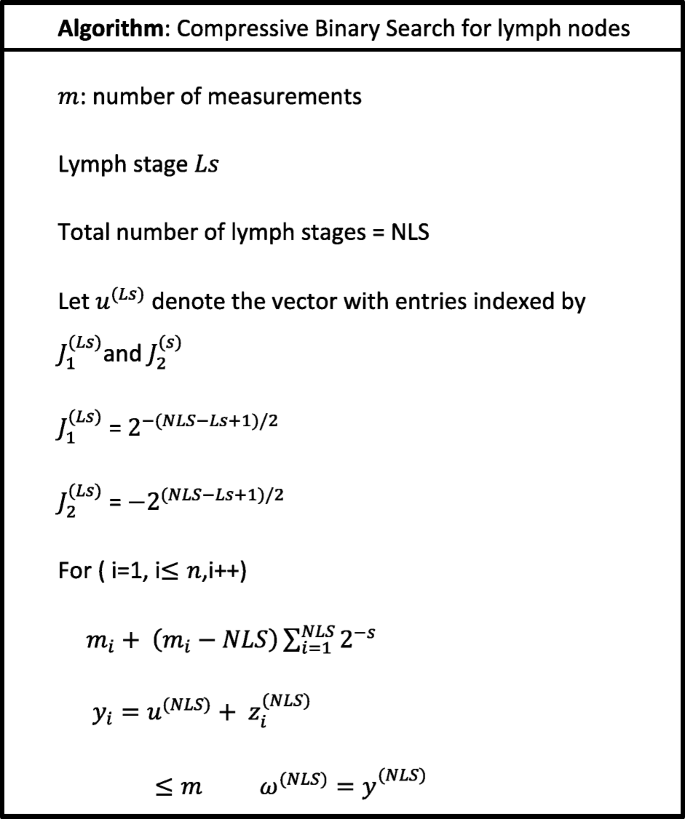
Ergebnisse und Diskussion
In order to analyze the proposed design, the nanoparticles were applied to the following five types of materials:silicone, lithium, lung, helium, and hydrogen. The materials were chosen because of their low density. The lung nanoparticles were samples from nano-sized lung nodules. They appear encircling with white shadows in a chest X-ray or computerized tomography scan taken from the lung of the person and required to be undamaged. The proposed idea is based on the analytical model, which indicates that the smaller the density of nanoparticles, the smaller the dynamic viscosity will be. This will result in a decrease in fluid viscosity. It is shown that the types of materials and the density of each particle will affect settling velocity of nanoparticles at entry into the lymphatic fluid and the density of the lymphatic fluid. We considered the following parameters:acceleration of gravity (g ) = 9.80665, particle diameter (d ) = 10 A, initial density of lymph fluid (ρf) = 998.28, and dynamic viscosity = 0.0010 kg m –1 s –1 [24]. These parameters were selected by the assumption that the viscosity of the lymphatic fluid is very similar to the viscosity of the water and the very small difference does not affect the results of the model. Figure 4 illustrates the density of nanoparticles for five selected materials for application in the proposed analytical model. Figure 5 shows the settling velocity for each particle. Figure 6 shows the effect of the settling velocity of nanoparticles on altering the lymphocyte density of cancer cells. The results shown in Fig. 6 show that the settling velocity of the particles carries a negative value. This indicates that the nanoparticle after entering in the lymphatic fluid rapidly moves in the opposite direction toward their entry into the lymphatic fluid. In general, any object that moves in the negative direction has a negative velocity. This movement of the particle leads to reduced viscosity of the lymphatic fluid.
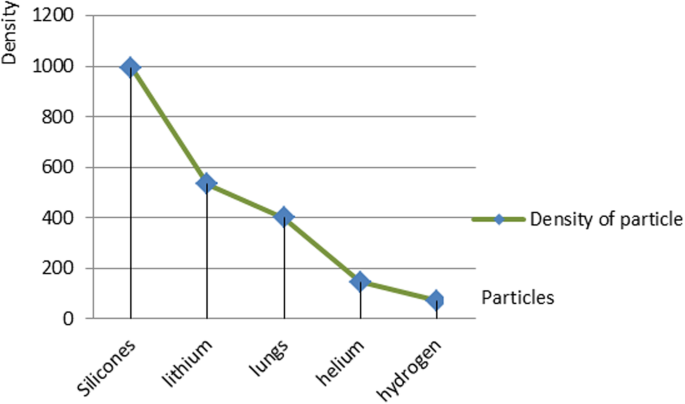
Density of nanoparticles for the five selected materials
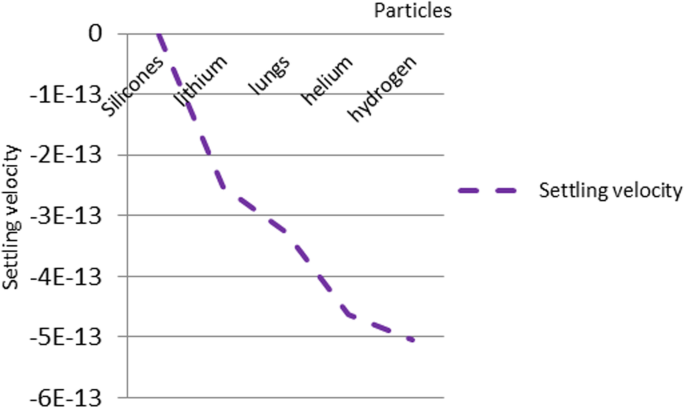
The settling velocity data for each particle
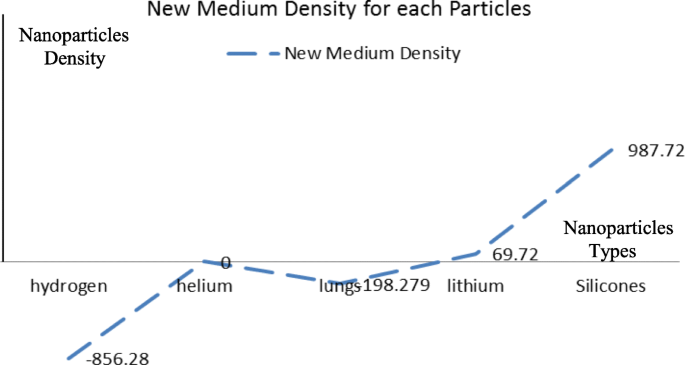
The effect of the settling velocity of nanoparticles on changing the lymphocyte density of cancer cells
Silicone nanoparticles showed the settling velocity of approximately − 2.87 × 10–15 m/s. This resulted in a decrease in viscosity of the lymphatic fluid to 987.72 kg/m 3 for the initial density 998.28 kg/m 3 . The density is continuously reduced to a point where hydrogen produces extremely spectacular results, i.e., the complete collapse of lymphatic fluid resistance. The density of the lymphatic fluid − 856.28 kg/m 3 with the negative sign indicated that there was no resistance from the lymphatic fluid to the flow of the nanoparticles, resulting in the complete collapse of the liquid fluid. Both the hydrogen and helium particles have a significant impact on the liquid viscosity due to the low density of the particles. Hence, it is important to use a drug system consisting of a group of nanoparticles for low-density materials. Figure 7 shows the relationship between the diameters of lung nanoparticles and the number of nanoparticles in one group. The figure shows that the higher the diameter of nanoparticles, the fewer their number in a group. This is clearly shown at the highest value of the nanoparticle diameter of 1000 nm, where the number of molecules in a group is 20 molecules. Figure 8 shows the relationship between the diameters of lithium nanoparticles and the number of nanoparticles in one group. This figure demonstrates the inverse relationship between the radius of nanoparticles and the number of molecules in a group where lithium particle diameters are significantly lower than the lung nanoparticles, where the number of nanoparticles in Fig. 7 is relatively low compared to the lithium particles as shown in Fig. 8. And the multicolor balls in both figures refer to different ranges of nanoparticle radii for each group, where each group contains a number of nanoparticles with different sizes. The best results can be obtained when hydrogen and helium particles are increased from other substances. A mixture of different materials should be used so that the properties of these substances can be used in the treatment process as well as to reduce viscosity. Figure 9 illustrates the different sets of materials proposed to have the mean highest density of both hydrogen and helium materials. Figure 10 shows the average mass of a nanoparticle in a group. It can be seen that the mass of both hydrogen and helium is the highest compared to the mass of particles of other substances. Figure 11 illustrates the relationship between the diameters of the nanoparticles and the width of its group or class. It is important to note that these results will open up a new area to reduce the resistance of the lymphatic fluid in tumors. This can be achieved using hydrogen nanoparticles of a size in the range of Angstrom. In addition to hydrogen nanoparticles, there may also exist a number of other substances in the same size. Figure 12 illustrates the standard deviation of a number of coefficients for both lung and lithium nanoparticles. These coefficients are limited to fractions of nanoparticles in a single group as well as their number in addition to the diameters of these nanoparticles. It is clear that the group fractions have the less value of the standard deviation. Hence, most of the fractions in the computational processes are around the mean of these values. Figure 13 shows the standard deviation of the mass for particles of silicones, lithium, lungs, helium, and hydrogen in one group. It is clear that the particles of the lung have the largest standard deviation and the lithium has the minimum value.
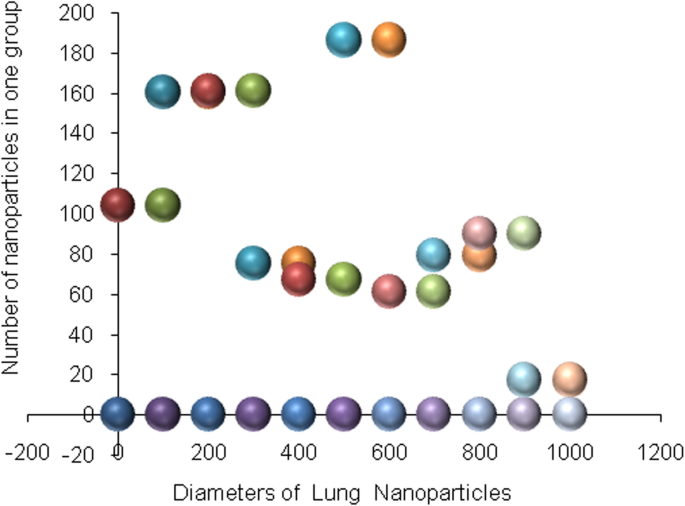
Group of nanoparticles in the lung cells and their number in one of the proposed groups
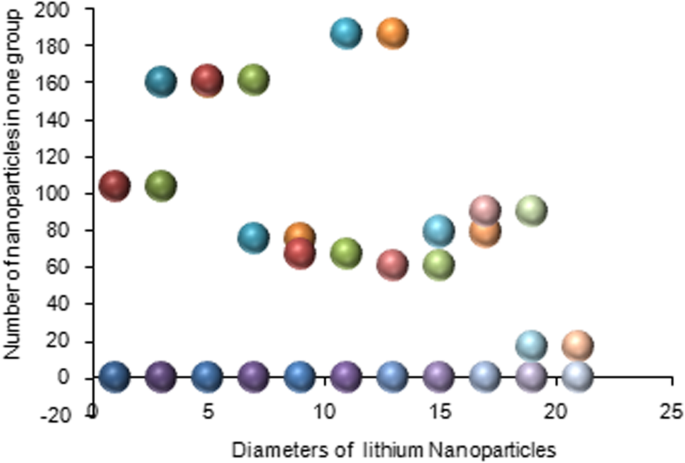
Group of nanoparticles in the lithium cells and their number in one of the proposed groups
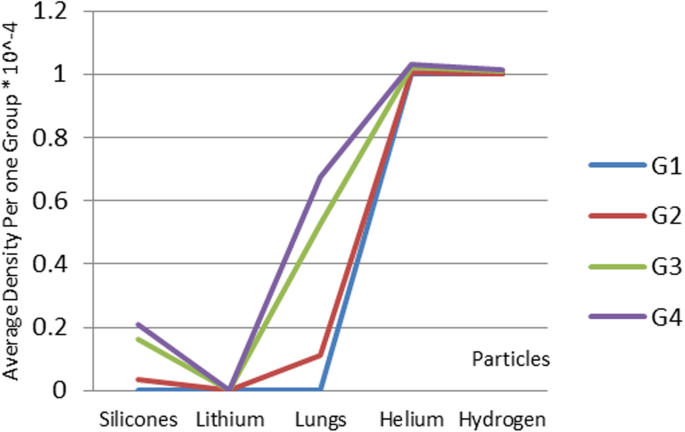
Different sets of materials proposed to have the mean highest density of both hydrogen and helium materials
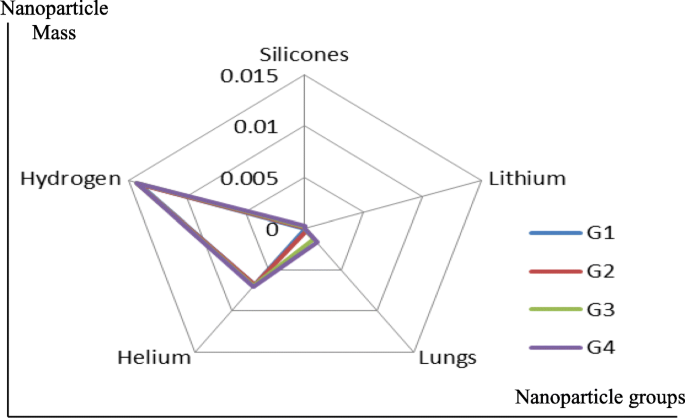
Average mass of a nanoparticle in a group
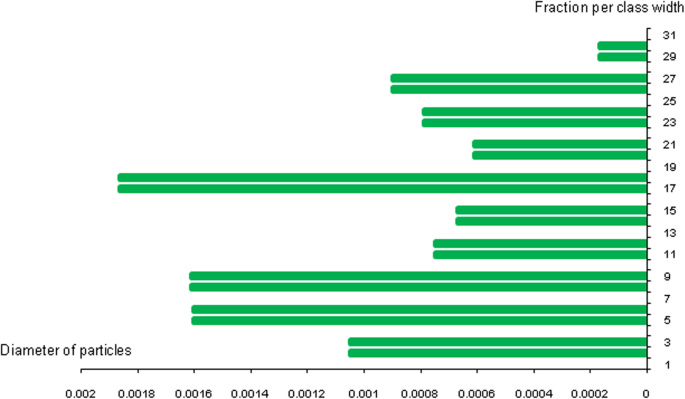
Diameters of the nanoparticles related to the group width
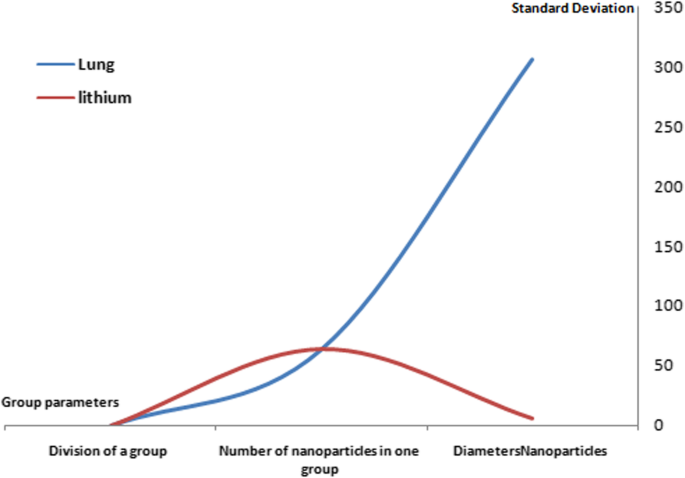
The standard deviation of lung and lithium nanoparticles coefficients
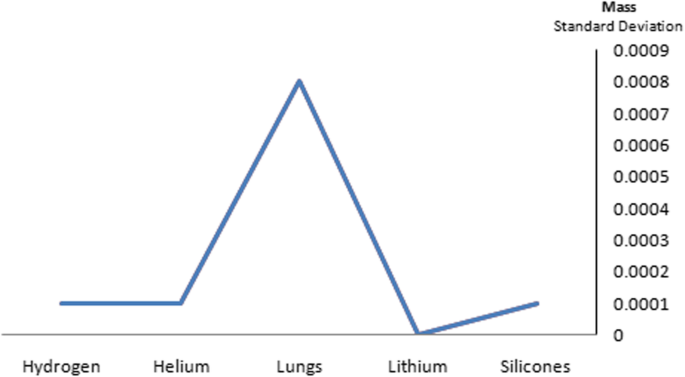
The standard deviation of the mass for particles of silicones, lithium, lungs, helium, and hydrogen in one group
Methods
The aim of this study was to establish a nano-drug delivery system capable of delivering the drugs effectively to the cancer cells. The following methodology was used to deliver nanoparticles:
- i)
Low-density nanoparticles
This study proposed the theoretical approach of nanoparticles as a low-density drug. This depends on the density and the settling velocity of the nanoparticles, as these nanoparticles can overcome the resistance of the lymphatic fluid.
- ii)
Preparation of anaerobic nanoparticles
This study uses the idea of nanoparticles possessing an antenna through which a connection can be made between nanoparticles and nano-controller. The transmission distance was assumed to be too small to match the composition of nanoparticles and also to fit the actual distance between them.
- iii)
Nano-controller design
Its function is to deliver the nanoparticle drug to cancer cells. Its role is to send signals to the nanoparticles and coordinate their actions and direct them to the lymphatic fluid of tumors.
- iv)
Searching for the target lymphatic nodes
The lymphatic nodes are searched using compressive binary search algorithm. This algorithm is characterized by high-speed search, which makes nanoparticles more accessible to infected cells than the conventional methods. The primary supervisor behind the performance of the nanoparticles is the nano-controller. It directs nanoparticles to the infected cells by following this algorithm to ensure that an appropriate number of molecules are in proportional density to the lymphatic fluid.
Schlussfolgerung
There have been various studies managing the treatment of malignant growth utilizing nanoparticles. The lymphatic liquid in tumors plays a substantial role in the obstruction of medication to the cancer cells. We developed an intelligent drug delivery system containing a consortium of nanoparticles. The proposed design demonstrates that small nanoparticles result in low density of the fluid. The results indicated that hydrogen particles are most efficient in reducing resistance toward lymphatic liquid owing to their smaller size. Furthermore, the design involves an anaerobic nano-controller that can determine the state and area of the particles. This technique conveys the medication to the infected cell more effectively.
Verfügbarkeit von Daten und Materialien
The datasets supporting the results of this article are included within the article.
Abkürzungen
- LN:
-
Lipid nanoparticles
- NLC:
-
Nanostructured lipid carriers
- PN:
-
Polymeric nanoparticles
- SLNs:
-
Solid lipid nanoparticles
Nanomaterialien
- Ausfallsicheres Design
- Nanofasern und Filamente für eine verbesserte Wirkstoffabgabe
- Biokompatible FePO4-Nanopartikel:Wirkstofftransport, RNA-Stabilisierung und funktionelle Aktivität
- Umweltverträgliche Metall-organische Gerüste als Wirkstoffabgabesystem für die Tumortherapie
- Zellbasierte Arzneimittelabgabe für Krebsanwendungen
- Zebrafisch:Ein vielversprechendes Echtzeit-Modellsystem für die nanotechnologisch vermittelte neurospezifische Wirkstoffabgabe
- 131I-gespurte PLGA-Lipid-Nanopartikel als Wirkstoffträger für die gezielte chemotherapeutische Behandlung von Melanomen
- Die Untersuchung eines neuartigen, durch Nanopartikel verbesserten wurmähnlichen Mizellensystems
- Nanotechnologie:vom In-vivo-Bildgebungssystem zur kontrollierten Medikamentenabgabe
- Zielgerichtete Endothelzellen mit multifunktionalen GaN/Fe-Nanopartikeln